Total scalp irradiation using pencil beam scanning proton therapy for dermatofibrosarcoma protuberans: case study and photon dosimetric comparison—a case report
Highlight box
Key findings
• Total scalp irradiation (TSI) using intensity-modulated proton therapy (IMPT) is dosimetrically favorable with unique technical considerations.
What is known and what is new?
• Modern photon therapy techniques such as intensity-modulated radiation therapy, volumetric-modulated arc radiotherapy, and helical tomotherapy have replaced electron therapy offering superior conformity and uniformity to treat irregular and concave total scalp surfaces while sparing underlying organs at risks (OARs) without the need for electron field matching.
• IMPT can achieve further highly conformal target dose distributions and OAR sparing utilizing its Bragg peak to create a sharp dose fall off at the distal end of the beam range.
What is the implication, and what should change now?
• IMPT achieved the best OAR sparing of the normal brain, hippocampus, and optic apparatus. Reduction of intracranial OARs dose is particularly appealing for young patients with good prognoses, who are expected to live for decades at risk for late toxicities.
Introduction
Background
Several diseases including dermatofibrosarcoma (1,2), angiosarcoma (3,4), non-melanomatous skin cancers, and cutaneous lymphoma (5,6) can involve scalp and facial tissue. For extensive disease, surgical resection when feasible followed by total scalp irradiation (TSI) is an optimal approach to eradicate the primary tumour. However, TSI presents technical difficulty and requires complex planning, owing to the large and irregular convex shape of the scalp target volume and the adjacent critical organs at risks (OARs) including the normal brain, globes, and lenses.
Rationale and knowledge gap
Different radiation therapy techniques have been studied and implemented to address such challenges. In the era of 3D-planned radiotherapy (3DRT), multiple electron fields without or with photon fields are used to conform the dose toward the entire scalp. When field matching is utilized, feathering techniques are often necessary to mitigate cold and hot spots. Able et al. (7) reported a 6-field electron technique with 2cm-shift field feathering, but they still saw dose variations near field junction (−10% to +50%). Akazawa (8) and Tung et al. (9) described a mixed technique utilizing 2 lateral electron fields and parallel opposed lateral photon fields, with improved dose uniformity. Modern techniques have replaced electron therapy in favor of pure intensity-modulated radiation therapy (IMRT), volumetric-modulated arc radiotherapy (VMAT), and helical tomotherapy (HT) photon plans. These techniques can offer superior conformity and uniformity to treat irregular and concave total scalp surfaces while sparing underlying OARs without the need for electron field matching, which are susceptible to setup errors and dose inhomogeneities (5,10,11). HT in particular is capable of providing tangential beams with no need for field matching (10). The dosimetric comparison studies of HT to 3DRT and VMAT by Song et al. (12) and Wojcicka et al. (13) found that HT provided the best conformality and the lowest mean brain dose among the photon-based techniques.
Intensity-modulated proton therapy (IMPT) can also achieve highly conformal target dose distributions and OAR sparing utilizing its Bragg peak to create a sharp dose fall off at the distal end of the beam range. In addition, proton therapy does not require bolus due to the relative lack of skin-sparing effect compared to photons. To date, there are few case reports describing the use of IMPT for TSI of the face and scalp. Sharma et al. (14) and Mizuno et al. (15) compared IMPT to VMAT and HT and reported that IMPT achieved the best OAR sparing of the normal brain, hippocampus, and optic apparatus. Reduction of intracranial OARs dose is particularly appealing for young patients with good prognoses, who are expected to live for decades at risk for late toxicities.
Objective
In this report, we will describe the planning and treatment of a clinical case who presented with dermatofibrosarcoma protuberans involving the majority of the scalp and treated with TSI using IMPT. We analysed the treatment robustness considering setup and range uncertainties and dose calculation accuracy, and we also created a coverage equivalent VMAT plan for dosimetric comparison. We present this article in accordance with the CARE reporting checklist (available at https://tro.amegroups.com/article/view/10.21037/tro-22-20/rc).
Case presentation
This case study was conducted under institutional review board (IRB) approval. All procedures performed in this study involving human participants were in accordance with the ethical standards of the institutional and/or national research committee(s) and with the Helsinki Declaration (as revised in 2013). Written informed consent was obtained from the patient for publication of this case report. A copy of the written consent is available for review by the editorial office of this journal.
Clinical case description
A 33-year-old female with history of head trauma as a child presented with a left frontal scalp lesion. For several years, the lesion was slowly progressive and further workup was not pursued. During her first pregnancy, however, the lesion rapidly progressed and became painful. Following delivery, she sought medical care. Biopsy confirmed dermatofibrosarcoma protuberans, grade 2, 18 mitoses/10 high-power field (HPF), and CD34 positive. Brain magnetic resonance imaging (MRI) demonstrated an irregular plaque-like enhancing lesion primarily involving the left frontoparietal scalp with posterior extension to the vertex and inferiorly to the temporal lobe. The lesion involved the dermis and subcutaneous tissues with overlying skin ulceration and in total measured. The tumor was inseparable from the pericranium but demonstrated no calvarial erosion or intracranial extension. Positron emission tomography (PET)/CT demonstrated fluorodeoxyglucose (FDG)-avid scalp thickening without underlying bone involvement or distant metastases.
She subsequently underwent a wide local excision of the primary lesion and two satellite nodules with a rotational free flap reconstruction. Pathology showed dermatofibrosarcoma protuberans, grade 1, with positive anterior, medial, and deep margins, no bone invasion, no LVI, and 1 mitosis/10 HPF, and CD34 positive. The main specimen measured as well as two satellite nodules each measuring. Postoperatively she healed well but continued to have left frontal pain along the suture line as well as expected cosmetic dissatisfaction.
The patient was referred to the New York Proton Center for consideration of postoperative proton beam therapy. A multidisciplinary tumor board reviewed her case and determined that the patient was not a candidate for further surgical resection considering the extensive positive margins. Systemic therapy was also not recommended.
The patient subsequently underwent postoperative proton therapy to a total of 6,000 cGy in 30 fractions. She developed expected grade 2 alopecia and grade 1 dermatitis and she had no weight loss throughout her treatment course. At 1-month follow up her left frontal pain improved significantly, and her skin irritation healed. At 3-month follow up, surveillance brain MRI demonstrated stable postoperative changes with no nodular pathologic enhancement and no T2/fluid-attenuated inversion recovery (FLAIR) hyperintensities in normal brain parenchyma. At 7-month follow-up, she reported stable left frontal suture pain and new regrowth of hairs along the treated area, however cosmesis remained unsatisfactory.
Proton beam therapy dose and volumes
Computed tomography (CT) and MRI simulation was performed for treatment planning. The patient was immobilized in a 5-point head and neck mask and deformable neck cushion. Intravenous contrast was administered for the planning MRI scan. The preoperative MRI scan was also fused with the simulation CT to delineate the preoperative extent of disease.
Two target volumes were identified. The clinical target volume (CTV)_5000 was defined as the postoperative bed with a 3-cm modified margin. The CTV_6000 was defined as the preoperative disease with a 3-cm modified margin. Treatment volume margins extended into the skin flap but the skin flap was not directly targeted. The CTV_5000 was treated to 5,000 cGy in 25 fractions (200 cGy/fraction). The CTV_6000 was then sequentially treated to an additional 1,000 cGy in 5 fractions (200 cGy/fraction) for a cumulative dose of 6,000 cGy.
Treatment Planning
The delivered IMPT plan was generated in the Eclipse treatment planning system (version 16.1, Varian Medical Systems, Palo Alto, CA, USA) using 4 superior oblique noncoplanar fields. Beam angles were chosen to deliver the dose distribution across the superior hemisphere as evenly as possible (Figure 1). A field-specific target (FST) was created for each field so that the TPS optimized dose delivery to the closest proximal CTV region without trespassing underlying OARs to cover the contralateral CTV. Sufficient overlap at junctional areas was maintained by manually adjusting the fields and beam arrangements to generate a slow dose gradient at field edge and therefore mitigate potential cold and hot spots due to setup errors (16). The multi-field optimized plan was generated using robust planning (Nonlinear Universal Proton Optimizer, version 16.1) (17) with 3 mm setup and 3.5% range uncertainties. Given the superficial nature of the target (~1.3 cm maximum depth), a 5-cm range shifter was used to improve target coverage and finer layer spacing (~2.5 mm) was incorporated into optimization to improve dose homogeneity and robustness. The final plan achieved a uniform and conformal dose distribution with 95% of prescription dose covering 99% of target volume and low OAR doses (Figure 2 and Table 1).
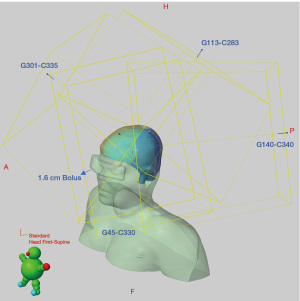
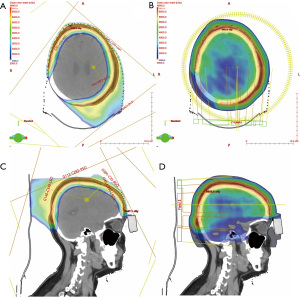
Table 1
OAR | Metric | IMPT | VMAT |
---|---|---|---|
Brain | Dmax (cGy) | 5,808 | 6,069 |
Dmean (cGy) | 453 | 2,885 | |
Temporal lobe_L | Dmean (cGy) | 1,066 | 3,679 |
Temporal lobe_R | Dmean (cGy) | 20 | 1,607 |
Hippocampus_L | Dmean (cGy) | 16 | 2,499 |
Hippocampus_R | Dmean (cGy) | 0 | 1,544 |
BrainStem | Dmax (cGy) | 5 | 2,223 |
Pituitary | Dmean (cGy) | 0 | 1,742 |
Chiasm | Dmax (cGy) | 3 | 1,792 |
Eye_L | Dmax (cGy) | 502 | 1,177 |
Eye_R | Dmax (cGy) | 135 | 1,055 |
Lens_L | Dmax (cGy) | 87 | 805 |
Lens_R | Dmax (cGy) | 5 | 823 |
Optic nerve_L | Dmax (cGy) | 10 | 1,519 |
Optic nerve_R | Dmax (cGy) | 4 | 1,275 |
Cochlea_L | Dmean (cGy) | 94 | 2,369 |
Cochlea_R | Dmean (cGy) | 0 | 1,079 |
OAR, organ at risk; IMPT, intensity-modulated proton therapy; VMAT, volumetric-modulated arc radiotherapy; Dmax, dose maximum; Dmean, dose mean; Gy, Gray; L, left; R, right.
In addition, a 2-cm customized bolus was placed on top of the eyes (Figure 1) without directly blocking the entrance beam of any field to further decrease the maximum dose of left eye, achieving a reduction in maximum dose to 501 cGy compared to 672 cGy without the bolus.
A comparison VMAT plan was generated using 2 full arcs of 6-MV X-ray beams with a 1.0 cm-thick bolus to achieve equivalent coverage of the PTV. Doses to all OARs were greater for the VMAT plan compared to the delivered IMPT plan, which is shown in the Table 1.
Plan verification
Initial attempt for planning using 4 coplanar fields was not acceptable due to significant coverage loss at skull vertex area, identified via Monte Carlo quality assurance (QA) check (18). This was caused by the known dose calculation inaccuracy of the analytical algorithms for tangential proton beams at tissue-air interfaces (19). Alternatively, the non-coplanar plan showed <3% D95% coverage degradation with Monte Carlo QA. Similarly, the new plan robustness evaluation using a 3-mm isocenter shifts and 3.5% range uncertainty showed a worst-case scenario for CTV coverage of D95% =93%, whereas the worst-case CTV coverage of the coplanar plan was D95% =78%.
Daily image guidance radiation therapy (IGRT) was performed with daily kV and weekly cone-beam computed tomography (CBCT). In vivo dosimetry measurement was performed for the first 2 treatments using OSLD dosimeter, which showed acceptable agreement (~6% less) with prescribed dose according to our institutional guidelines. CT verification scans were obtained every other week to evaluate the delivered dose distribution and robustness. One replan was implemented at week 3 to minimize dose to the uninvolved hair-bearing region at the posterior nape of neck.
Discussion
In the current case study, TSI with IMPT provided excellent target coverage while significantly sparing underlying OARs, such as normal brain tissue and optical structures. A comparison VMAT plan was generated to quantify the absolute and relative differences in OAR doses. In particular, IMPT reduced the mean dose to the normal brain parenchyma and hippocampus compared to VMAT by over 84% and 99%, respectively. These results are appealing for young patients, such as in this case, who have a favorable prognosis and are expected to be at risk of developing neurocognitive deficits and/or secondary malignancies for decades. Other prominent OARs with major dose reductions were the bilateral globes (>78% mean dose reduction), lenses (>89% max dose reduction), and cochlea (>97% mean dose reduction). Mean pituitary dose was also essentially eliminated from 1,742 to 0.3 cGy. The dose reductions identified in our study were consistent with a prior case report comparing IMPT and VMAT for TSI of a nonmelanoma skin cancer patient described by the Maryland Proton Treatment Center (14). A separate dosimetric study similarly reported improved OAR sparing with IMPT compared to HT, which is a photon-based system particularly suited for scalp irradiation due to its ability to deliver tangential beams in a continuous fashion (12). In combination, these studies suggest that IMPT consistently reduces overall integral dose, particularly to brain parenchyma, compared to modern photon-based modalities.
Unique technical challenges were identified during the IMPT treatment planning process, including the limitation of coplanar fields in providing reliable coverage of the vertex scalp, and the limitation of standard TPS algorithms to account for shallow air-tissue interfaces for tangential proton beams. Our robustness analysis and Monte Carlo comparison showed that optimal en-face and non-trespassing midline beam arrangements are key to fully utilizing the advantage of proton therapy to achieve optimal dose distributions. The Mayo Clinic similarly reported the need for custom Monte Carlo optimization for reliable dose calculations for TSI plans (20). A customized bolus was also utilized to further minimize any scattering dose delivered to the eyes and lenses.
Another important consideration includes the range uncertainty and robustness evaluation, particularly at overlapping field junctions. Close attention should be paid to verification scans and OSLD measurements to confirm expected dose deposition, especially in the absence of Monte Carlo planning. Our experience supports the use of overlapping field junction gradients to mitigate the impact of setup error on dose homogeneity. Of note, photon-based techniques using tangential fields can also be sensitive to setup uncertainty affecting target coverage, and therefore routine IGRT is recommended for all TSI cases (13).
Conclusions
In conclusion, this case study demonstrates the feasibility of IMPT to treat a complex concave target while minimizing dose to underlying OARs. There are novel technical concerns with this technique, however, and attentive physics and dosimetry expertise are required for safe and reliable delivery.
Acknowledgments
Funding: This research was funded in part through the NIH/NCI Cancer Center Support (No. P30 CA008748).
Footnote
Provenance and Peer Review: This article was commissioned by the editorial office, Therapeutic Radiology and Oncology for the series “Pencil Beam Scanning Particle Therapy”. The article has undergone external peer review.
Reporting Checklist: The authors have completed the CARE reporting checklist. Available at https://tro.amegroups.com/article/view/10.21037/tro-22-20/rc
Conflicts of Interest: All authors have completed the ICMJE uniform disclosure form (available at https://tro.amegroups.com/article/view/10.21037/tro-22-20/coif). The series “Pencil Beam Scanning Particle Therapy” was commissioned by the editorial office without any funding or sponsorship. C.C.C. served as an unpaid editorial board of Therapeutic Radiology and Oncology from June 2021 to May 2023 and served as the unpaid Guest Editor of the series. Shaakir Hasan received payment for PTCOG conference attendance from NYPC. H.L. received payment from Varian and declared the topic FLASH Bragg peak delivery from NYPC physics team. J.I.C. received payment from Varian Medical Systems for speaker honorarium. A.M.C. received consulting fees and honorarium from Icotec and Dyn’R Medical Systems. C.B.S. II received payment from Varian Medical Systems for honorarium. R.H.P. is the unpaid Radiation Oncology Section Chair of New York Roentgen Society. The authors have no other conflicts of interest to declare.
Ethical Statement: The authors are accountable for all aspects of the work in ensuring that questions related to the accuracy or integrity of any part of the work are appropriately investigated and resolved. This case study was conducted under institutional review board (IRB) approval. All procedures performed this study involving human participants were in accordance with the ethical standards of the institutional and/or national research committee(s) and with the Helsinki Declaration (as revised in 2013). Written informed consent was obtained from the patient for publication of this case report. A copy of the written consent is available for review by the editorial office of this journal.
Open Access Statement: This is an Open Access article distributed in accordance with the Creative Commons Attribution-NonCommercial-NoDerivs 4.0 International License (CC BY-NC-ND 4.0), which permits the non-commercial replication and distribution of the article with the strict proviso that no changes or edits are made and the original work is properly cited (including links to both the formal publication through the relevant DOI and the license). See: https://creativecommons.org/licenses/by-nc-nd/4.0/.
References
- Mendenhall WM, Zlotecki RA, Scarborough MT. Dermatofibrosarcoma protuberans. Cancer 2004;101:2503-8. [Crossref] [PubMed]
- Ballo MT, Zagars GK, Pisters P, et al. The role of radiation therapy in the management of dermatofibrosarcoma protuberans. Int J Radiat Oncol Biol Phys 1998;40:823-7. [Crossref] [PubMed]
- Guadagnolo BA, Zagars GK, Araujo D, et al. Outcomes after definitive treatment for cutaneous angiosarcoma of the face and scalp. Head Neck 2011;33:661-7. [Crossref] [PubMed]
- Hata M, Wada H, Ogino I, et al. Radiation therapy for angiosarcoma of the scalp: treatment outcomes of total scalp irradiation with X-rays and electrons. Strahlenther Onkol 2014;190:899-904. [Crossref] [PubMed]
- Samant RS, Fox GW, Gerig LH, et al. Total scalp radiation using image-guided IMRT for progressive cutaneous T cell lymphoma. Br J Radiol 2009;82:e122-5. [Crossref] [PubMed]
- Mellenberg DE, Schoeppel SL. Total scalp treatment of mycosis fungoides: The 4 × 4 technique. Int J Radiat Oncol Biol Phys 1993;27:953-8. [Crossref] [PubMed]
- Able CM, Mills MD, McNeese MD, et al. Evaluation of a total scalp electron irradiation technique. Int J Radiat Oncol Biol Phys 1991;21:1063-72. [Crossref] [PubMed]
- Akazawa C. Treatment of the scalp using photon and electron beams. Med Dosim 1989;14:129-31. [Crossref] [PubMed]
- Tung SS, Shiu AS, Starkschall G, et al. Dosimetric evaluation of total scalp irradiation using a lateral electron-photon technique. Int J Radiat Oncol Biol Phys 1993;27:153-60. [Crossref] [PubMed]
- Orton N, Jaradat H, Welsh J, et al. Total scalp irradiation using helical tomotherapy. Med Dosim 2005;30:162-8. [Crossref] [PubMed]
- Casto Z, Liu M, Lenards N, et al. Multiple case dosimetric evaluation of VMAT scalp irradiation using 3D milled bolus. Med Dosim 2021;46:324-7. [Crossref] [PubMed]
- Song JH, Jung JY, Park HW, et al. Dosimetric comparison of three different treatment modalities for total scalp irradiation: the conventional lateral photon-electron technique, helical tomotherapy, and volumetric-modulated arc therapy. J Radiat Res 2015;56:717-26. [Crossref] [PubMed]
- Wojcicka JB, Lasher DE, McAfee SS, et al. Dosimetric comparison of three different treatment techniques in extensive scalp lesion irradiation. Radiother Oncol 2009;91:255-60. [Crossref] [PubMed]
- Sharma AM, Kowalski E, McGovern N, et al. Proton Versus Intensity-Modulated Radiation Therapy: First Dosimetric Comparison for Total Scalp Irradiation. Int J Part Ther 2020;6:19-26. [Crossref] [PubMed]
- Mizuno T, Tomita N, Takaoka T, et al. Dosimetric Comparison of Helical Tomotherapy, Volumetric-Modulated Arc Therapy, and Intensity-Modulated Proton Therapy for Angiosarcoma of the Scalp. Technol Cancer Res Treat 2021;20:1533033820985866. [Crossref] [PubMed]
- Lin H, Ding X, Kirk M, et al. Supine craniospinal irradiation using a proton pencil beam scanning technique without match line changes for field junctions. Int J Radiat Oncol Biol Phys 2014;90:71-8. [Crossref] [PubMed]
- Stützer K, Lin A, Kirk M, et al. Superiority in Robustness of Multifield Optimization Over Single-Field Optimization for Pencil-Beam Proton Therapy for Oropharynx Carcinoma: An Enhanced Robustness Analysis. Int J Radiat Oncol Biol Phys 2017;99:738-49. [Crossref] [PubMed]
- Huang S, Kang M, Souris K, et al. Validation and clinical implementation of an accurate Monte Carlo code for pencil beam scanning proton therapy. J Appl Clin Med Phys 2018;19:558-72. [Crossref] [PubMed]
- Huang S, Souris K, Li S, et al. Validation and application of a fast Monte Carlo algorithm for assessing the clinical impact of approximations in analytical dose calculations for pencil beam scanning proton therapy. Med Phys 2018;45:5631-42. [Crossref] [PubMed]
- Hunzeker A, Mundy DW, Ma J, et al. Intensity-Modulated Proton Therapy (IMPT) Treatment of Angiosarcoma of the Face and Scalp. Int J Part Ther 2021;8:304-10. [Crossref] [PubMed]
Cite this article as: Huang S, Jang E, Lin H, Chen CC, Hasan S, Choi JI, Chhabra AM, Simone CB 2nd, Press RH. Total scalp irradiation using pencil beam scanning proton therapy for dermatofibrosarcoma protuberans: case study and photon dosimetric comparison—a case report. Ther Radiol Oncol 2023;7:23.