Retrospective analysis of dosimetry on hippocampal-avoidance whole-brain radiotherapy in a regional hospital
Introduction
Brain metastases are the most common intracranial tumors in adults. They pose a significant cause of morbidity and mortality, affecting approximately 10 % to 30 % of adult cancer patients (1). The increased incidence of brain metastasis can be attributed to a variety of factors, including an aging population, improved systemic treatment, and improved imaging methods for detecting smaller brain metastases in asymptomatic patients. For patients with multiple brain metastases, whole-brain radiotherapy (WBRT) has been the standard palliative treatment (1,2). Prior to the 21st century, patients with brain metastases had a poor prognosis. With treatment, the overall median survival after diagnosis is approximately 4–6 months; no long-term cognitive deficits were observed after WBRT. With the advent of targeted therapies and advanced treatments, cancer patients with brain metastases can now live for longer periods of time, even for years after WBRT (3). Although the most important benefit of WBRT is control of metastatic brain lesions, neurocognitive decline may occur in patients with longer survival (4,5).
The neural stem cell compartment, which is located in the subgranular zone of the hippocampal dentate gyrus, has been linked to memory formation. Hippocampal neural stem cell injury during WBRT may play a crucial role in neurocognitive decline (5-9). According to the Radiation Therapy Oncology Group (RTOG) 0933 trial, hippocampal-avoidance (HA)-WBRT may reduce radiation-induced neurocognitive toxicities (5,6). Confirmed HA-WBRT in Phase II or III trial could have preserved better cognitive function without the increase in intracranial progression-free survival or overall survival, and should be considered standard of care for patients with no metastases in the HA region (7,8). According to Ghia et al., the incidence of metastases within 5 mm of the parahippocampal region is low. Thus, their findings suggested that a 5-mm margin around the hippocampus for HA-WBRT was an acceptable risk (9).
HA-WBRT presents a significant technical challenge in treatment planning in order to spare the hippocampus, which is located in the center of the brain and completely surrounded by the planning target volume (PTV). RTOG 0933 recommends strict dose criteria; it requires a high-level homogeneity and precise radiation delivery. Numerous studies on this subject apply complex treatment planning to deliver an adequate coverage, such as helical TomoTherapy or the linear accelerator (Linac)-based intensity-modulated radiation therapy (IMRT) technique (10-17). Further development of the sophisticated volumetric-modulated arc therapy (VMAT) technique allows for fractionated HA-WBRT (13,15-17). Gondi et al. discovered that modern radiation therapy techniques, whether helical TomoTherapy or Linac-based treatment plans, all allow for hippocampus sparing with acceptable target coverage and homogeneity (12), and Nevelsky et al. claimed that Elekta therapy machine can achieve Linac-based VMAT for HA-WBRT treatment (14).
However, this presents a major challenge on clinical workload with the time-consuming HA-WBRT treatment. Thus, the treatment could deliver HA-WBRT at the cost of noncompliance and non-conformity of dose distribution on PTV, even deviations from the guideline on clinical scenario. Because the radiation treatment plan is a documented source in radiation therapy, retrospective quality evaluation is needed to identify inconsistencies of dosimetry parameters. In this study, we intend to evaluate the plan quality and dosimetry in delivering HA-WBRT in a regional hospital retrospectively. We present the following article in accordance with the STROBE reporting checklist (available at https://tro.amegroups.com/article/view/10.21037/tro-22-17/rc).
Methods
Study population
We retrospectively analyzed cancer patients with brain metastases who were diagnosed at a regional referral hospital in Tainan, Taiwan, between January 2014 and December 2020. We reviewed the medical records of patients who received HA-WBRT. The following data were extracted from the medical database: age, gender, histology, radiotherapy plan, and dosimetry parameters. Patients who had previously received brain irradiation or who did not complete HA-WBRT were excluded, while patients eligible for WA-WBRT were over the age of 20, had a fair-to-good performance status with an Eastern Cooperative Oncology Group score of ≤2.
In addition, patients underwent brain magnetic resonance imaging (MRI) to rule out more than four metastatic foci, or patients with a single lesion were surgically resected or suited to stereotactic radiosurgery. Brain metastasis detected less than 5 mm of perihippocampal region were also excluded. The study was conducted in accordance with the Declaration of Helsinki (as revised in 2013). The study was approved by Institutional Review Board, Chi Mei Medical Center, Liouying (IRB No. 10603-L06) and informed consent was taken from all the patients.
Treatment planning and delivery
All enrolled patients underwent a computed tomography simulation scan of the entire head with a 2-mm slice thickness, using a thermoplastic mask to immobilize them. Before HA-WBRT, all patients should have had a brain MRI to delineate the bilateral hippocampi; the delineation was established and confirmed by an experienced radiation oncologist. HA regions are created by three-dimensionally expanding hippocampal contours by 5 mm to allow for a sharp dose fall-off. The whole-brain parenchyma is defined as the clinical tumor volume (CTV). The PTV is defined as the CTV minus the HA regions and plus a margin to allow for geometrical uncertainty. The Pinnacle (Philips, Fitchburg, WI, USA) or TomoTherapy (Accuray, Sunnyvale, CA, USA) planning systems were used to generate plans for 6-MV photon beams. Pinnacle 3 Version 14 was used to optimize all 6-MV VMAT plans for Elekta Synergy, with 40 multi-leaf collimator leaf pairs of 1 cm leaf width at the isocenter. Our previous study reported on the detailed planning technique (15). Treatment parameters for TomoTherapy patients were treated with a slice width of 1.05 cm, a modulation factor with a mean of 2.5 (range, 2.5–3) and a pitch with a mean of 0.287 (range, 0.287–0.3). The prescribed dose was 30 Gy in 10–12 fractions by physician’s preference.
Dosimetry analyses of HA-WBRT across different brain structures
The following parameters were evaluated for the organs at risk and PTV: PTV V30Gy, PTVD2%, PTV D98%, Dmax of the hippocampus, and Dmax of the optic chiasm. The following are the RTOG 0933 compliance criteria for HA-WBRT (target and normal tissue planning doses): At least 95% of the PTV receives 30 Gy (V30Gy >95% PTV), 2% of the PTV receives 37.5 Gy or less (D2% ≤37.5 Gy), 98% of the PTV receives 25 Gy or more (D98% PTV ≥25 Gy), the minimum dose to the hippocampi (Dmin=D100%) was 10 Gy, the maximum dose to the hippocampi was 17 Gy (5,6).
Deviation from the HA-WBRT plan was defined as when the dose parameters are in the protocol’s unacceptable deviation column. Unacceptable deviations include PTV V30Gy <90% (less than 90% of PTV received at least 30 Gy), D2% of PTV >40 Gy, hippocampus Dmax >17 Gy, Dmin >10 Gy, and maximum dose of optic nerve or chiasm >37.5 Gy (5,6).
Delivery time comparison
The time needed to deliver a single fraction of HA-WBRT was recorded. The delivery time is defined as the time elapsed between the first beam-on and the last beam-off, excluding the patient setup and daily imaging procedures. Treatment delivery time was measured during delivery of the calculated plans.
Statistical analysis
The clinical features and dosimetry parameters were described in detail. The Wilcoxon/Mann-Whitney test or Fisher’s exact test were used to evaluate corresponding variables. SPSS (Version 24.0). Armonk, NY: IBM Corp.) was used for all analyses. The two-tailed significance level was set at 0.05.
Results
There were 21 eligible cancer patients with brain metastasis who underwent HA-WBRT. Table 1 shows the demographic and clinical data of cancer patients. At the time of referral for brain irradiation, the patients’ ages ranged from 36 to 81 years (median, 58 years). Ten patients (48%) were male. Fourteen patients (67%) had lung cancer, six (28%) had breast cancer, and one (5%) had liver cancer. There were 50 brain metastatic lesions in total. The median number of metastases was 2 (range, 1–4). Sixteen patients (76%) received Linac-based VMAT treatment, while five accepted TomoTherapy. There was no patient who received upfront neurosurgical resection. Extracranial disease was clinically controlled in 13 patients (62%).
Table 1
Characteristics | Patients (N=21) |
---|---|
Age (years), median [range] | 58 [36–81] |
Gender, n [%] | |
Man | 10 [48] |
Woman | 11 [52] |
Performance (ECOG), n [%] | |
0–1 | 14 [67] |
2 | 7 [33] |
Histologic type of primary tumor, n [%] | |
Lung, NSCLC | 13 [62] |
Lung, SCLC | 1 [5] |
Breast | 6 [28] |
Liver | 1 [5] |
Number of brain metastasis at diagnosis, n [%] | |
1 | 8 [38] |
2 | 4 [19] |
3 | 2 [10] |
4 | 7 [33] |
Status of extracranial metastasis, n [%] | |
Controlled | 13 [62] |
Not controlled | 8 [38] |
Neurosurgery before radiotherapy, n [%] | |
Yes | 0 [0] |
No | 21 [100] |
Radiotherapy modality, n [%] | |
Linac-based VMAT | 16 [76] |
TomoTherapy | 5 [24] |
Linac, linear accelerator; NSCLC, non-small cell lung cancer; SCLC, small cell lung cancer; VMAT, volumetric-modulated arc therapy.
Dosimetry evaluation of brain organs showed that the volume of the hippocampi ranged from 2.3 to 11.5 mL (median, 5 mL), the volume of the HA ranged from 15.2 to 76.9 mL (median, 35.1 mL), and the volume of the whole-brain parenchyma ranged from 1,205 to 1,614 mL (median, 1,369 mL). (V30Gy PTV) coverage ranged from 69% to 96%, with a median of 85%. The volume of the PTV V25Gy ranged from 85% to 100% (median, 96.6%). The maximal dose to bilateral hippocampi ranged from 9.2 to 25.8 Gy, with a median of 14.4 Gy (Table 2).
Table 2
Characteristics | Patients (N=21) |
---|---|
Hippocampus volume (mL), median (range) | 5.0 (2.3−11.5) |
HA volume (mL), median (range) | 35.1 (15.2−76.9) |
Brain volume (mL), median (range) | 1,369 (1,205−1,614) |
HA volume/brain volume (%) (range) | 2.5 (1.2−5.4) |
PTV (V30Gy) coverage (%), median [range] | 85 [69−96] |
PTV (V25Gy)a (%), median [range] | 96.6 [85−100] |
Dmax of hippocampus (Gy), median (range)b | 14.4 (9.2−25.8) |
a, volume of PTV receive dose more than 25 Gy; b, maximum dose on hippocampus. HA, hippocampal-avoidance; PTV, planning target volume.
Table 3 shows the dosimetry analysis of pairwise comparisons between Linac-based VMAT therapy and TomoTherapy. An example of the isodose lines (ranging from 30, 25, to 16 Gy) around the hippocampus are displayed in Figure 1. When PTV (V30Gy>95%) coverage was compared across modalities, Linac-based VMAT planning was comparable to TomoTherapy counterpart, with only one plan deviating from RTOG 0933 criteria (6% vs. 20%, P=0.43). Both treatments met the RTOG 0933 criteria in hot spots (D2 % ≤37.5 Gy) evaluation. In terms of the minimum PTV dose criteria (D98 % >25 Gy), TomoTherapy (4/5, 80%) outperformed Linac VMAT treatment (3/16, 19%) (P=0.03). In terms of the constraint on both hippocampi (D100% <10 Gy), Linac-based VMAT had nine plans (9/16, 56%) that were unable to meet the protocol’s criteria, as opposed to TomoTherapy, which all met the protocol; however, this difference did not achieve statistical significance (P=0.1).
Table 3
Structure and dosimetry metrics (RTOG 0933 protocol criteria) | Non-compliance with criteria, n [%] | P valuea | |
---|---|---|---|
Linac-based (n=16) | TomoTherapy (n=5) | ||
Planning target volume | |||
V30Gy >95% | 1 [6] | 1 [20] | 0.43 |
D2% ≤37.5 Gy | 16 [100] | 5 [100] | 1 |
D98% >25 Gy | 3 [19] | 4 [80] | 0.03* |
Hippocampus | |||
D100% (Dmin) <10 Gy | 9 [56] | 5 [100] | 0.1 |
Dmax <17 Gy | 11 [69] | 4 [80] | 0.55 |
Optic nerves & chiasm | |||
Dmax <37.5 Gy | 16 [100] | 5 [100] | 1 |
Approximate delivery time (sec) | 364 | 437 | 0.03* |
a, P value for Wilcoxon/Mann-Whitney or Fisher’s exact test; *, P<0.05. RTOG, Radiation Therapy Oncology Group.
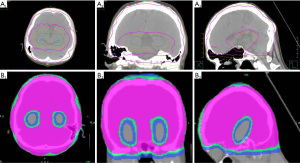
When comparing the maximum doses for optic nerves and chiasm (Dmax <37.5 Gy), both Linac-based VMAT and TomoTherapy all achieve required constraint. When it came to therapy delivery time, TomoTherapy took the longest (median: 437 sec), averaging over 5 patients, compared to 364 sec for Linac VMAT averaging over 16 patients, achieving a significant statistical difference (P=0.03).
Discussion
WBRT can provide rapid relief of neurologic symptoms and improve quality of life, which is especially beneficial in patients whose brain metastases are surgically inaccessible or when the patient is unable to undergo neurosurgery (1,2). Patients with limited intracranial disease are recommended focal therapeutic options, such as neurosurgical resection or stereotactic radiosurgery, to prevent risks of cognitive deterioration and decline in learning and recall function following WBRT (2,4). In order to preserve neurocognitive function, the HA-WBRT technique could be an option in brain metastasis without hippocampal involvement (5-9).
RTOG 0933 was a prospective phase II trial designed to confirm the efficacy of HA-WBRT in preserving neurocognitive function (5,6). Another phase II trial conducted in Taiwan by Yang et al. confirmed no differences in intracranial progression and overall survival with better memory preservation, suggesting that HA-WBRT could be recommended as a standard of care for brain metastatic patients with good performance status and no metastasis in the HA region (8). Following these prospective clinical trials to confer neuro-cognitive protection in metastatic brain disease, HA-WBRT has gained clinical acceptance (7,8). However, perihippocampal failure has been reported from clinical observations (18-20). We have also reported unusual cases of intracranial failure following HA-WBRT therapy and perihippocampal failure rate was about 8% in our previous report (18).
RTOG 0933 recommended stringent dose criteria which required a high dose homogeneity and precise radiation delivery. The protocol imposed strict limits on PTV coverage as well as the dose to organs at risk. However, plan optimization of the HA-WBRT is a complex process, including a steep gradient between minimal dose to the hippocampus, homogeneous target coverage, and maximal dose to the PTV, so multiple iterations are usually required before all constraints are met. The manual or automated optimization process, as well as the standardization of high-level plan quality, takes time (10-17). Gondi et al. published a “how-to” guide for achieving conformal hippocampal sparing with helical TomoTherapy and Linac-based IMRT therapy (12). In our previous study, we found that non-coplanar arcs outperformed coplanar arcs in terms of D2% ≤37.5 Gy and D98% >25 Gy (P<0.05), as well as similar homogeneity and conformity between coplanar and non-coplanar planning on HA-WBRT (15). In this study, PTV V30Gy coverage ranged from 69% to 96% (median, 85%), and PTV V25Gy volume ranged from 85% to 100% (median, 97%). Based on the findings, we hypothesize that increasing the number of arc and using a non-coplanar VMAT technique could result in better dose PTV coverage, but it would also lengthen the treatment time (15-17).
The volumes of bilateral hippocampi range from 2.3 to 11.5 mL with a median volume of 5 mL in our study. Nonetheless, the delineation was established by residents and confirmed by radiation oncologists, we also regarded it seem a relative high volume of hippocampus in some contouring in this retrospective analysis. Contouring of the hippocampi can be challenging and a RTOG contouring atlas was published to aid in their delineation, however, the disparity on manual contours by the radiation oncologists had arguable in the clinical scenario (12,15-17,21). In patients who received either Linac VMAT or helical TomoTherapy, the median dose to the hippocampi was 14.4 Gy, and the maximum dose was 25.8 Gy, both of which were classified as an unacceptable deviation. All reports in the literature have shown excellent results in sparing the hippocampi of HA-WBRT planning (10-17). However, review of the literature, most studies compared re-calculated treatment plan from patient’s image dataset rather than clinical quality analysis of planning rounds in radiation therapy. In our clinical scenario, we also took extra care to keep the left hippocampus consistent per protocol if the patient only had right temporal lobe metastasis. According to Kazda et al., studies have shown that unilateral sparing of the dominant (left) hippocampus during WBRT may be able to mitigate the cognitive decline, particularly verbal memory, similar to the widely studied bilateral HA-WBRT (22). Le Fèvre et al. reported shielding at least one hippocampus by delivering the lowest possible dose is recommended, so that cognitive function can be preserved (23). The dilemma does exist in balancing clinical workload with the time-consuming planning required to meet all criteria, so daily treatment may come at the expense of noncompliance and non-conformity on planning targets, even with some deviations from RTOG 0933 (24).
The results of pair comparison with Linac-based VMAT and TomoTherapy did not show statistically significant differences in criteria among PTV coverage (V30Gy), and D2% hot spot, indicating that the performance of Linac-based VMAT is comparable to TomoTherapy. The coverage of D98% of TomoTherapy outperforms Linac-based VMAT with statistical significance (P=0.03). Gondi et al. demonstrated that helical TomoTherapy outperformed Linac-based IMRT in terms of PTV coverage and homogeneity, so this difference can be attributed to the faster dose fall-off provided by helical TomoTherapy (12). Furthermore, TomoTherapy appears to be superior to Linac-based VMAT in terms of minimizing Dmin <10 Gy dose in the hippocampus region (100% vs. 56%). However, probable due to limited case numbers, we were unable to detect the significance of these parameters in this study, whereas the literature Linac-based IMRT or VMAT techniques all reported for hippocampus sparing with acceptable target coverage and homogeneity (10-17).
The average delivery time of Linac-based VMAT was faster than TomoTherapy (364 vs. 437 sec, P=0.03). Furthermore, Rong et al. reported a 15-minute delivery time for Linac-based IMRT and an 18-minute delivery time for TomoTherapy. Thus, the relatively slow delivery time in TomoTherapy (more than 8–15 min on average) can be attributed to the smaller jaw width’s narrow collimation (25). In a previous study of Linac-based VMAT, we found that average delivery times were 289 sec (coplanar) and 372 sec (non-coplanar) (15). In Taiwan, patients with brain metastasis cancer are only reimbursed by the National Health Insurance system for palliative setting. Thus, widespread use of HA-WBRT in routine radiotherapy is being conserved; the high cost of optimizing hippocampal planning is also not fully reimbursed.
Finally, due to the study’s retrospective nature and small sample size, we only demonstrated the introspective nature of the dosimetry analysis deviated from RTOG 0933 criteria in clinical settings. So the dilemma does exist in balancing clinical workload with the time-consuming planning required to meet all criteria, so daily treatment may come at the expense of noncompliance and non-conformity on planning targets, even with deviations from protocol. In determining the final plan, the individual choice of the physician according to the patient’s clinical situation may have played a role definitely. That is, in an actual clinical situation, other clinical factors may have been considered more priority than strict adherence to the RTOG 0933 criteria. The patient’s life expectancy or the location and size of the gross tumor would have been considered before selecting the final treatment plan. However, subsequent intra-cranial failure either caused by inadequate dosing coverage or only from aggressiveness of cancer per se awaits further study.
Acknowledgments
We thank Enago™ offering help in reviewing and revising the manuscript for grammar and syntax.
Funding: This article was supported by the Department of Clinical Research of Chi Mei Hospital, Liouying (No. CLFHR11108).
Footnote
Reporting Checklist: The authors have completed the STROBE reporting checklist. Available at https://tro.amegroups.com/article/view/10.21037/tro-22-17/rc
Conflicts of Interest: All authors have completed the ICMJE uniform disclosure form (available at https://tro.amegroups.com/article/view/10.21037/tro-22-17/coif). LTS and SYH report that this article was supported by the Department of Clinical Research of Chi Mei Hospital, Liouying (No. CLFHR11108). The other authors have no conflicts of interest to declare.
Ethical Statement:
Open Access Statement: This is an Open Access article distributed in accordance with the Creative Commons Attribution-NonCommercial-NoDerivs 4.0 International License (CC BY-NC-ND 4.0), which permits the non-commercial replication and distribution of the article with the strict proviso that no changes or edits are made and the original work is properly cited (including links to both the formal publication through the relevant DOI and the license). See: https://creativecommons.org/licenses/by-nc-nd/4.0/.
References
- Khuntia D, Brown P, Li J, et al. Whole-brain radiotherapy in the management of brain metastasis. J Clin Oncol 2006;24:1295-304. [Crossref] [PubMed]
- Gaspar LE, Mehta MP, Patchell RA, et al. The role of whole brain radiation therapy in the management of newly diagnosed brain metastases: a systematic review and evidence-based clinical practice guideline. J Neurooncol 2010;96:17-32. [Crossref] [PubMed]
- Miller JA, Kotecha R, Ahluwalia MS, et al. Overall survival and the response to radiotherapy among molecular subtypes of breast cancer brain metastases treated with targeted therapies. Cancer 2017;123:2283-93. [Crossref] [PubMed]
- Brown PD, Jaeckle K, Ballman KV, et al. Effect of Radiosurgery Alone vs Radiosurgery With Whole Brain Radiation Therapy on Cognitive Function in Patients With 1 to 3 Brain Metastases: A Randomized Clinical Trial. JAMA 2016;316:401-9. [Crossref] [PubMed]
- Gondi V, Tome WA, Marsh J, et al. Estimated risk of perihippocampal disease progression after hippocampal avoidance during whole-brain radiotherapy: safety profile for RTOG 0933. Radiother Oncol 2010;95:327-31. [Crossref] [PubMed]
- Gondi V, Pugh SL, Tome WA, et al. Preservation of memory with conformal avoidance of the hippocampal neural stem-cell compartment during whole-brain radiotherapy for brain metastases (RTOG 0933): a phase II multi-institutional trial. J Clin Oncol 2014;32:3810-6. [Crossref] [PubMed]
- Brown PD, Gondi V, Pugh S, et al. Hippocampal Avoidance During Whole-Brain Radiotherapy Plus Memantine for Patients With Brain Metastases: Phase III Trial NRG Oncology CC001. J Clin Oncol 2020;38:1019-29. [Crossref] [PubMed]
- Yang WC, Chen YF, Yang CC, et al. Hippocampal avoidance whole-brain radiotherapy without memantine in preserving neurocognitive function for brain metastases: a phase II blinded randomized trial. Neuro Oncol 2021;23:478-86. [Crossref] [PubMed]
- Ghia A, Tomé WA, Thomas S, et al. Distribution of brain metastases in relation to the hippocampus: implications for neurocognitive functional preservation. Int J Radiat Oncol Biol Phys 2007;68:971-7. [Crossref] [PubMed]
- Krayenbuehl J, Di Martino M, Guckenberger M, et al. Improved plan quality with automated radiotherapy planning for whole brain with hippocampus sparing: a comparison to the RTOG 0933 trial. Radiat Oncol 2017;12:161. [Crossref] [PubMed]
- Prokic V, Wiedenmann N, Fels F, et al. Whole brain irradiation with hippocampal sparing and dose escalation on multiple brain metastases: a planning study on treatment concepts. Int J Radiat Oncol Biol Phys 2013;85:264-70. [Crossref] [PubMed]
- Gondi V, Tolakanahalli R, Mehta MP, et al. Hippocampal-sparing whole-brain radiotherapy: a "how-to" technique using helical tomotherapy and linear accelerator-based intensity-modulated radiotherapy. Int J Radiat Oncol Biol Phys 2010;78:1244-52. [Crossref] [PubMed]
- Pokhrel D, Sood S, Lominska C, et al. Potential for reduced radiation-induced toxicity using intensity-modulated arc therapy for whole-brain radiotherapy with hippocampal sparing. J Appl Clin Med Phys 2015;16:131-41. [Crossref] [PubMed]
- Nevelsky A, Ieumwananonthachai N, Kaidar-Person O, et al. Hippocampal-sparing whole-brain radiotherapy using Elekta equipment. J Appl Clin Med Phys 2013;14:4205. [Crossref] [PubMed]
- Hung CL, Chang PS, Jong SB, et al. To investigate the treatment plan in hippocampal-sparing whole brain radiotherapy using linear accelerator-based volumetric modulated arc therapy. Therapeut Radiol Oncol 2018;25:121-8. [Crossref]
- Pokhrel D, Sood S, McClinton C, et al. Treatment planning strategy for whole-brain radiotherapy with hippocampal sparing and simultaneous integrated boost for multiple brain metastases using intensity-modulated arc therapy. Med Dosim 2016;41:315-22. [Crossref] [PubMed]
- Chen LJ, Li MH, Cheng HW, et al. Hippocampus-sparing whole-brain radiotherapy: dosimetric comparison between non-coplanar and coplanar planning. Ther Radiol Oncol 2021;5:1. [Crossref]
- Shieh LT, Lee SW, Chen CC, et al. Perihippocampal failure after hippocampal-avoidance whole-brain radiotherapy in cancer patients with brain metastases: Results of a retrospective analysis. Medicine (Baltimore) 2022;101:e29144. [Crossref] [PubMed]
- Yeo SG. Perihippocampal metastasis following hippocampus-avoiding prophylactic cranial irradiation for small cell lung cancer: a case report. Onco Targets Ther 2017;10:4017-21. [Crossref] [PubMed]
- Cho Y, Lee J, Lee IJ, et al. Intracranial failure after hippocampal-avoidance prophylactic cranial irradiation in limited-stage small-cell lung cancer patients. Sci Rep 2021;11:7435. [Crossref] [PubMed]
- Lo H, Estoesta P, Mackonis EC, et al. Clinical evaluation of RTOG-atlas based contouring, MRI-atlas auto-contouring, and neuroradiologist contouring of the hippocampi for hippocampal avoidance whole-brain radiotherapy. Ther Radiol Oncol 2021;5:19. [Crossref]
- Kazda T, Vrzal M, Prochazka T, et al. Left hippocampus sparing whole brain radiotherapy (WBRT): A planning study. Biomed Pap Med Fac Univ Palacky Olomouc Czech Repub 2017;161:397-402. [Crossref] [PubMed]
- Le Fèvre C, Cheng X, Loit MP, et al. Role of hippocampal location and radiation dose in glioblastoma patients with hippocampal atrophy. Radiat Oncol 2021;16:112. [Crossref] [PubMed]
- Bijdekerke P, Verellen D, Tournel K, et al. TomoTherapy: implications on daily workload and scheduling patients. Radiother Oncol 2008;86:224-30. [Crossref] [PubMed]
- Rong Y, Evans J, Xu-Welliver M, et al. Dosimetric evaluation of intensity-modulated radiotherapy, volumetric modulated arc therapy, and helical tomotherapy for hippocampal-avoidance whole brain radiotherapy. PLoS One 2015;10:e0126222. [Crossref] [PubMed]
Cite this article as: Yen CH, Shieh LT, Lee SW, Chen CC, Ho SY. Retrospective analysis of dosimetry on hippocampal-avoidance whole-brain radiotherapy in a regional hospital. Ther Radiol Oncol 2023;7:2.