Establish a comprehensive plan robustness evaluation platform for intensity modulated proton therapy for bilateral head and neck cancer based on the daily cone-beam computed tomography: a dose accumulation study between the PTV based IMPT (PTV-IMPT) and CTV based robust optimized IMPT (ro-IMPT) planning strategies
Introduction
Proton beam therapy (PBT) has been clinically used for several decades. More recently, due to decreasing cost, increasing availability, and improved technology, the adoption of proton therapy has been increasing throughout the world in the last ten years. In addition, with the advent of pencil beam scanning (PBS) technology, intensity modulated proton therapy (IMPT) is now possible and allows for improved conformality around irregular targets.
PBT has been of great interest in head and neck cancer (HNC) treatment which provides better normal tissue sparing such as parotid and oral cavity (OC), compared to photon radiotherapy (1-4). PBT is also commonly used in re-irradiation patients to mitigate the toxicity of the surrounding tissues (5-7). In the upfront definitive setting, multiple studies have demonstrated improved dosimetry and clinical toxicity of IMPT over photon treatments (1-4,8,9).
PBT is inherently sensitive to setup and range uncertainties. This problem is even more pronounced when IMPT is used (10,11). In addition, the weight loss experienced by HNC patients often results in significant dosimetric changes, which cannot be predicted using a standard physics model. Thus, it is important to use adaptive offline replanning to account for the changes (4,12). However, the optimal timing and interval are largely unknown, and it remains a resource-intensive task in a busy clinic due to these additional validation-CTs (vCTs). There is an immediate need to generate a workflow to assess the plan’s robustness daily and design a robust treatment planning protocol to ensure the plan quality throughout the treatment course.
Recently, cone-beam computed tomography (CBCT), which was successfully implemented on the proton gantry in the university of Pennsylvania since 2014 (13), has been considered as a new standard of clinical configuration in the new proton therapy center design, preparation, and installation. Besides excellent 3D volumetric image registration and correction, CBCT is able to assess a patient’s geometry change or deformation in each treatment fraction. However, due to CBCT technique limitations, the imaging quality in terms of imaging contrasts or inaccuracy CT Hounsfield unit (HU) is not as good as standard CT sim, therefore cannot be used for proton dose calculation directly. However, with the recent advancement in the CBCT imaging correction and synthetic-CT (sCT) generation algorithm (14-16), these corrected CBCT could be used directly for dose calculation to access the dose distribution daily. Such a feature is critical to the PBT for HNC.
Meanwhile, the planning strategy for bilateral HNC using IMPT is evolving from planning target volume (PTV)-based planning to a clinical target volume (CTV)-based robust optimized planning based on understanding the uncertainties and geometry changes (17). However, no study has quantitatively assessed the plan target coverage throughout the treatment course. To our best knowledge, this is the first study to integrate a comprehensive platform to validate the sCT dose calculation accuracy through bi-weekly vCT and quantitatively assess the dose accumulation throughout the treatment course for the two different IMPT planning strategies. We present the following article in accordance with the MDAR reporting checklist (available at https://tro.amegroups.com/article/view/10.21037/tro-21-42/rc).
Methods
IMPT planning strategies
Nine bilateral HNC patients who previously received 35 fractions volumetric modulated arc therapy (VMAT) in our institution were selected in this study which was approved by the institutional review board (#2017-455), and individual consent for this retrospective analysis was waived. Baseline patient characteristics are summarized in Table 1. All patients underwent CT simulation for planning and intravenous contrast for contour and concurrent chemotherapy for their definitive treatment. Thermoplastic facemasks were used for immobilization. Prophylactic gastronomy tubes were placed in all patients to prevent significant weight loss during the course of treatment. All patients had a daily CBCT obtained during their 35 treatments. Daily imaging guided radiation therapy (IGRT) shifts were recorded and used for daily dose calculation as well as to reproduce the daily setup and treatment delivery.
Table 1
Patients | Primary | Laterality | T stage | N stage |
---|---|---|---|---|
1 | Base of tongue | Right | T2 | N2b |
2 | Unknown | Right | T0 | N2b |
3 | Base of tongue | Right | T4 | N2c |
4 | Base of tongue | Left | T2 | N2b |
5 | Tonsil | Left | T2 | N1 |
6 | Base of tongue | Left | T2 | N2c |
7 | Base of tongue | Right | T2 | N2b |
8 | Base of tongue | Left | T1 | N2b |
9 | Base of tongue | Right | T1 | N2b |
Initial planning was done on the planning CT (pCT). Two planning strategies were used: (I) PTV-based (PTV-IMPT); and (II) CTV based robust optimized IMPT (ro-IMPT). All planning was done in Raystation™ version 5.02 (RaySearch Laboratories, Stockholm, Sweden). A 4-field setup with bilateral anterior and posterior oblique beams was used in both PTV-IMPT and ro-IMPT groups. For PTV-IMPT planning strategy, a PTV margin of 3 mm was used. For ro-IMPT, CTV was used as the optimization target with robust optimization parameter setting as 3 mm setup and 3.5% range uncertainties (total 21 scenarios). All gross tumor volumes (GTVs), CTVs, and organ at risks (OARs) were contoured and verified by a physician. CTV_high was prescribed 7,000 cGy (gross disease) and CTV_low/med was prescribed 6,000 cGy (subclinical disease) in thirty-five fractions. ro-IMPT group was normalized to CTVs V100 >98% on the pCT. In addition, the PTV-IMPT group ensured the PTV coverage received PTV V100 >98%. For the OARs optimization constraints, a maximum brainstem dose of 5,400 and 4,500 cGy for the spinal cord were respected. A maximum mandible dose of 7,000 cGy was used for planning. A mean parotid dose of 2,600 cGy was used for planning, and the mean dose was pushed as low as possible without sacrificing target coverage. Similarly, a mean OC dose of 3,400 cGy, mean larynx dose of 4,400 cGy and mean pharyngeal constrictor (PC) dose of 5,000 cGy was used for planning. Again, those structures were pushed as low as possible without sacrificing CTV coverage or increasing parotid dose.
sCT generation from CBCT
To generate the sCT, a research version of a commercial Deformable Image Registration (DIR) tool (ADMIRE 2.0, Elekta Inc., Stockholm, Sweden) was utilized for the DIR between CT and CBCT. Briefly, the intra-patient algorithm of ADMIRE performs a block-wise non-linear registration to get a robust initial alignment, followed by a dense local-correlation-coefficient (LCC) based deformable registration to get the final deformable vector field (DVF). This tool has been reported and evaluated in several international challenges of head & neck and lung patients DIR with high-ranking results (18-21). Our institution also comprehensively evaluated for HNC patients with expert-delineated contours as ground truth, including seven OARs [brain stem, cord, left and right (L/R) parotids, L/R submandibular gland and mandible] (22). The CT-CT intra-patient propagation achieved Dice similarity coefficient (DICE) greater than 0.85 and mean surface distance (MSD) smaller than 1.2 mm. The DICE and MSD of CT-CBCT propagation were very close to the CT-CT results, decreasing only by 0.03 and 0.2 mm respectively.
sCT validation test
To validate the sCT proton dose calculation accuracy, both vCT and sCT on the same day were used for comparison purpose for both PTV-IMPT and ro-IMPT groups.
Dose Accumulations and plan robustness analysis
In this study, the initial contours were deformed along with the images and manually checked by a physician for accuracy on the sCTs (14-16). Dose accumulation throughout the treatment course for each patient was obtained by summing up the dose recalculated on each daily sCT (total 35 sCTs throughout the treatment courses). Daily CBCT registration shifts from the previous VMAT on the linear accelerators (LINACs) were used to mimic the daily setup uncertainty in the dose recalculation.
Doses to target and normal structure were analyzed among the two planning strategies: PTV-IMPT and ro-IMPT. The difference in “real” dose accumulated (Dose-A) between the two planning groups was compared with the two-sided t-test. The study was conducted in accordance with the Declaration of Helsinki (as revised in 2013). The study was approved by institutional review board (No. 2017-455) and consent was waived from all individual participants.
Statistics analysis
A two-tailed Wilcoxon Signed Rank test were performed. Hypotheses and determine level of significance: H0 (the median difference is zero) versus H1 (the median difference is not zero α=0.05). With the example size, the critical value of W is 8.
Results
The sCT were compared to two vCTs on the same day with proton dose comparison. Using the 18 pairs of same day’s sCT and vCT for both PTV-IMPT and ro-IMPT plans, the average gamma index using 3%/3 mm on the entire volume showed a 98.2%±1.5% pass rate (Figure 1).
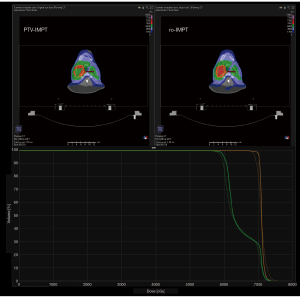
The dosimetric results are summarized in Table 2 (target coverage) and Table 3 (OARs). An example patient is shown in Figure 2A,2B. In the PTV-IMPT plan group, the mean V100 of CTV_high and CTV_low received 99.48% and 99.60% of the prescription dose in (initial dose) Dose-I. However, the Dose-A showed that the mean V100 of CTV_high and CTV_low were degraded to 80.70% and 85.73%, respectively, after 35 treatment fractions. As a comparison, in the ro-IMPT plan group, the mean V100 of CTV_high and CTV_low received 99.17% and 98.94% of the prescription dose in Dose-I. After 35 treatment fractions, the Dose-A showed that the mean V100 of CTV_high and CTV_low were degraded slightly to 96.72% and 96.13%, respectively. The mean accumulated dose difference between the ro-IMPT Dose-A vs. PTV-IMPT Dose-A plans for CTV_high/CTV_low was 16.33%/11.06% (we have statistically significant evidence at α=0.05) showed a superior advantage of using ro-IMPT planning strategy for target robustness coverage in bilateral HNC proton treatment.
Table 2
Patient | Planning method | CTV1 V100 initial (%) | CTV1 V100 accumulated (%) | CTV2 V100 initial (%) | CTV2 V100 accumulated (%) |
---|---|---|---|---|---|
1 | PTV-IMPT | 99.50 | 66.97 | 99.44 | 93.88 |
ro-IMPT | 98.69 | 96.84 | 98.14 | 98.81 | |
2 | PTV-IMPT | 100.00 | 84.88 | 99.48 | 88.68 |
ro-IMPT | 99.17 | 92.12 | 98.80 | 87.79 | |
3 | PTV-IMPT | 99.51 | 89.25 | 99.66 | 92.96 |
ro-IMPT | 99.09 | 99.23 | 98.69 | 97.78 | |
4 | PTV-IMPT | 99.48 | 84.84 | 99.86 | 84.40 |
ro-IMPT | 98.73 | 98.93 | 99.16 | 96.08 | |
5 | PTV-IMPT | 98.47 | 96.72 | 99.74 | 92.10 |
ro-IMPT | 98.60 | 97.75 | 98.99 | 98.68 | |
6 | PTV-IMPT | 99.03 | 88.05 | 99.29 | 67.80 |
ro-IMPT | 99.44 | 95.94 | 98.38 | 95.30 | |
7 | PTV-IMPT | 99.54 | 72.18 | 99.19 | 85.77 |
ro-IMPT | 99.28 | 95.31 | 99.16 | 97.12 | |
8 | PTV-IMPT | 100.00 | 63.24 | 99.93 | 79.36 |
ro-IMPT | 99.83 | 96.78 | 99.20 | 98.49 | |
9 | PTV-IMPT | 99.78 | 80.19 | 99.78 | 86.61 |
ro-IMPT | 99.71 | 97.61 | 99.97 | 95.11 | |
Mean | PTV-IMPT | 99.48 | 80.70 | 99.60 | 85.73 |
ro-IMPT | 99.17 | 96.72 | 98.94 | 96.13 |
CTV, clinical target volume; PTV, planning target volume; IMPT, intensity modulated proton therapy; ro-IMPT, robust optimized IMPT.
Table 3
OAR | Planning method | Initial plan, mean [range] | Accumulated plan, mean [range] |
---|---|---|---|
Brainstem max (cGy) | PTV-IMPT | 1,939 [703–2,840] | 2,093 [1,052–2,714] |
ro-IMPT | 1,809 [933–2,406] | 1,999 [1,286–2,682] | |
Spinal cord max (cGy) | PTV-IMPT | 2,004 [1,236–3,258] | 2,516 [1,283–3,761] |
ro-IMPT | 2,204 [1,489–3,349] | 2,586 [1,433–3,655] | |
Ipsilateral parotid mean (cGy) | PTV-IMPT | 2,943 [2,104–3,761] | 3,272 [2,101–4,276] |
ro-IMPT | 2,829 [2,213–3,465] | 3,178 [1,903–3,994] | |
Contralateral parotid mean (cGy) | PTV-IMPT | 1,397 [862–1,990] | 1,814 [1,167–2,697] |
ro-IMPT | 1,362 [983–2,075] | 1,802 [1,242–2,979] | |
Mandible D0.1cc (cGy) | PTV-IMPT | 6,964 [6,867–7,007] | 6,986 [6,708–7,227] |
ro-IMPT | 6,993 [6,865–7,151] | 7,014 [6,704–7,132] | |
Larnx mean (cGy) | PTV-IMPT | 4,662 [3,037–6,152] | 5,030 [3,327–6,565] |
ro-IMPT | 4,674 [3,100–6,245] | 4,997 [3,331–6,288] | |
Oral cavity mean (cGy) | PTV-IMPT | 3,391 [2,102–4,335] | 3,694 [2,720–4,703] |
ro-IMPT | 3,501 [2,630–4,917] | 3,749 [2,736–5,407] | |
Constrictor mean (cGy) | PTV-IMPT | 5,854 [5,289–6,266] | 6,003 [5,377–6,451] |
ro-IMPT | 5,699 [5,152–6,129] | 5,869 [5,234–6,385] | |
Constrictor V70 Gy (%) | PTV-IMPT | 23.3 [0–43.2] | 23.9 [10.9–36.5] |
ro-IMPT | 19.7 [0–39.2] | 21.86 [0–40.6] |
OAR, organ at risk; PTV, planning target volume; IMPT, intensity modulated proton therapy; ro-IMPT, robust optimized IMPT.
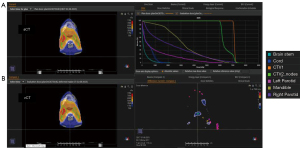
The mean accumulated dose for ipsilateral and contralateral parotid was 32.7 and 18.1 Gy, respectively. The mean maximum accumulated dose for the brainstem and spinal cord was 20.9 and 25.2 Gy, respectively. Given that no robustness optimization was placed on the OARs, there was no difference in the dose to the OAR with the different planning methods. Based on the final dose accumulation, none of the OARs received unacceptable doses to critical organs (spinal cord, brainstem, and mandible) in either planning group.
We also examined the average daily variation in coverage and compared the two planning strategies throughout these nine HNC patients (Figure 3). The PTV-IMPT showed a continued degradation of coverage throughout the treatment course. While the ro-IMPT also showed decreasing coverage through the treatment course, its degradation is significantly less than that of the PTV-IMPT method.
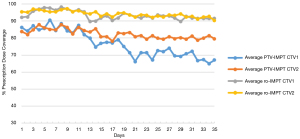
There was one patient (#2) with suboptimal coverage (CTV_V100 <95%) with the ro-IMPT planning methods. The patient experienced a 4 kg weight loss over the treatment course that likely contributed to the suboptimal coverage.
Discussion
Clinically, proton therapy remains an attractive option for upfront definitive cases due to the dosimetric advantage and potential to decrease toxicity. Unfortunately, there is a lack of robust data regarding clinical improvement with proton therapy compared to photon therapy. A case-matched analysis from MD Anderson Cancer Center (MDACC) showed a decreased weight loss or G-tube use with IMPT over intensity-modulated radiation therapy (IMRT). Initially, improved G2−3 xerostomia at 3 months did not persist at 1 year (9). In addition, MDACC also published the patient-reported outcomes (PRO) on a similar cohort of the patient population that showed improvement of IMPT with subacute food taste and appetite and chronic appetite over IMRT. Overall though, there was little difference in PRO in the two groups (8). One of the main advantages of proton therapy is the sparing of contralateral parotids compared to photons, and we would anticipate xerostomia to improve with proton therapy. The lack of improvement in xerostomia may be due to their planning method, as it seems there is no significant dose difference in the parotid mean dose based on their prior planning publications (3). Further prospective trials focusing on toxicity are needed to determine the utility of proton therapy. At this time, there is an accruing multi-institutional randomized trial comparing IMRT vs. IMPT for HNC with the primary outcome measuring late grade 3–5 toxicity (NCT01893307). To ensure the accurate dose to be delivered to the HNC patients, multiple planning strategies were proposed, including robust optimization.
This is the first quantitative and comprehensive clinical study to assess the treatment robustness via these two planning strategies using daily CBCT throughout the whole treatment course. A number of dosimetric studies have demonstrated decreased dose to OAR with IMPT compared to photon IMRT (1-4). However, due to the physical properties of proton therapy, the final dose given to the patient may not be representative based on the initial plan. Proton is inherently more susceptible to motion and uncertainties than photon (23,24). Our results are encouraging and show that ro-IMPT is a valid planning strategy to treat patients with IMPT. On the other hand, PTV-IMPT is a non-robust method, indicating that it may not be the best option for HNC patient treatment in the clinic as the prior study also demonstrates worse coverage with PTV-IMPT (17). Eight out of ten patients had CTV >95% coverage with ro-IMPT.
One patient in this study for which ro-IMPT performed sub-optimally and may be attributed to weight loss during the treatment course. There have been several studies in the past that examine the issue of weight loss in HNC patients and found that critical weight loss during treatment is extremely common (30–50%) (25,26). Multiple patient factors such as pre-treatment body mass index (BMI), age, primary site, dose, chemotherapy, etc. have been shown to predict weight loss. However, for IMPT, it remains critically important to monitor the patient’s weight during treatment and have an established protocol for re-imaging and adaptive replanning if the weight loss effects dosimetry. Most importantly, it is critical to perform adaptive replanning at certain time intervals as described by other groups (4,27,28). The optimal time point remains debated. However, this study established a comprehensive platform that gives clinicians data to determine the optimal time point to perform the proton adaptive plans. Based on the data in this study (Figure 3), a good starting time point for adaptation maybe every 2–2.5 weeks with weekly evaluation CT to encompass the majority of patients that may have major changes in anatomy.
Conclusions
With this daily proton dose evaluation platform, we established a system for comprehensive robustness evaluation platform into our daily workflow to evaluate the need for replanning taking weight and anatomy changes into account. The study found that the ro-IMPT strategy achieves superior and more robust target coverage than PTV-IMPT in bilateral HNC treatment. Special cautions are needed to take into account the significant weight changes.
Acknowledgments
Funding: This study, in part, was supported by the research seed grant award from Beaumont Health.
Footnote
Provenance and Peer Review: This article was commissioned by the editorial office, Therapeutic Radiology and Oncology, for the series “Pencil Beam Scanning Particle Therapy”. The article has undergone external peer review.
Reporting Checklist: The authors have completed the MDAR reporting checklist. Available at https://tro.amegroups.com/article/view/10.21037/tro-21-42/rc
Data Sharing Statement: Available at https://tro.amegroups.com/article/view/10.21037/tro-21-42/dss
Conflicts of Interest: All authors have completed the ICMJE uniform disclosure form (Available at https://tro.amegroups.com/article/view/10.21037/tro-21-42/coif). The series “Pencil Beam Scanning Particle Therapy” was commissioned by the editorial office without any funding or sponsorship. XD received honorarium fee from IBA speaker Bureau outside the work presented here. The authors have no other conflicts of interest to declare.
Ethical Statement: The authors are accountable for all aspects of the work in ensuring that questions related to the accuracy or integrity of any part of the work are appropriately investigated and resolved. The study was conducted in accordance with the Declaration of Helsinki (as revised in 2013). The study was approved by institutional review board (No. 2017-455) and consent was waived from all individual participants.
Open Access Statement: This is an Open Access article distributed in accordance with the Creative Commons Attribution-NonCommercial-NoDerivs 4.0 International License (CC BY-NC-ND 4.0), which permits the non-commercial replication and distribution of the article with the strict proviso that no changes or edits are made and the original work is properly cited (including links to both the formal publication through the relevant DOI and the license). See: https://creativecommons.org/licenses/by-nc-nd/4.0/.
References
- van de Water TA, Lomax AJ, Bijl HP, et al. Potential benefits of scanned intensity-modulated proton therapy versus advanced photon therapy with regard to sparing of the salivary glands in oropharyngeal cancer. Int J Radiat Oncol Biol Phys 2011;79:1216-24. [Crossref] [PubMed]
- Apinorasethkul O, Kirk M, Teo K, et al. Pencil beam scanning proton therapy vs rotational arc radiation therapy: A treatment planning comparison for postoperative oropharyngeal cancer. Med Dosim 2017;42:7-11. [Crossref] [PubMed]
- Holliday EB, Kocak-Uzel E, Feng L, et al. Dosimetric advantages of intensity-modulated proton therapy for oropharyngeal cancer compared with intensity-modulated radiation: A case-matched control analysis. Med Dosim 2016;41:189-94. [Crossref] [PubMed]
- Simone CB 2nd, Ly D, Dan TD, et al. Comparison of intensity-modulated radiotherapy, adaptive radiotherapy, proton radiotherapy, and adaptive proton radiotherapy for treatment of locally advanced head and neck cancer. Radiother Oncol 2011;101:376-82. [Crossref] [PubMed]
- Romesser PB, Cahlon O, Scher ED, et al. Proton Beam Reirradiation for Recurrent Head and Neck Cancer: Multi-institutional Report on Feasibility and Early Outcomes. Int J Radiat Oncol Biol Phys 2016;95:386-95. [Crossref] [PubMed]
- Phan J, Sio TT, Nguyen TP, et al. Reirradiation of Head and Neck Cancers With Proton Therapy: Outcomes and Analyses. Int J Radiat Oncol Biol Phys 2016;96:30-41. [Crossref] [PubMed]
- McDonald MW, Zolali-Meybodi O, Lehnert SJ, et al. Reirradiation of Recurrent and Second Primary Head and Neck Cancer With Proton Therapy. Int J Radiat Oncol Biol Phys 2016;96:808-19. [Crossref] [PubMed]
- Sio TT, Lin HK, Shi Q, et al. Intensity Modulated Proton Therapy Versus Intensity Modulated Photon Radiation Therapy for Oropharyngeal Cancer: First Comparative Results of Patient-Reported Outcomes. Int J Radiat Oncol Biol Phys 2016;95:1107-14. [Crossref] [PubMed]
- Blanchard P, Garden AS, Gunn GB, et al. Intensity-modulated proton beam therapy (IMPT) versus intensity-modulated photon therapy (IMRT) for patients with oropharynx cancer - A case matched analysis. Radiother Oncol 2016;120:48-55. [Crossref] [PubMed]
- Kraan AC, van de Water S, Teguh DN, et al. Dose uncertainties in IMPT for oropharyngeal cancer in the presence of anatomical, range, and setup errors. Int J Radiat Oncol Biol Phys 2013;87:888-96. [Crossref] [PubMed]
- Soukup M, Söhn M, Yan D, et al. Study of robustness of IMPT and IMRT for prostate cancer against organ movement. Int J Radiat Oncol Biol Phys 2009;75:941-9. [Crossref] [PubMed]
- Góra J, Kuess P, Stock M, et al. ART for head and neck patients: On the difference between VMAT and IMPT. Acta Oncol 2015;54:1166-74. [Crossref] [PubMed]
- Veiga C, Janssens G, Teng CL, et al. First Clinical Investigation of Cone Beam Computed Tomography and Deformable Registration for Adaptive Proton Therapy for Lung Cancer. Int J Radiat Oncol Biol Phys 2016;95:549-59. [Crossref] [PubMed]
- Landry G, Nijhuis R, Dedes G, et al. Investigating CT to CBCT image registration for head and neck proton therapy as a tool for daily dose recalculation. Med Phys 2015;42:1354-66. [Crossref] [PubMed]
- Kurz C, Dedes G, Resch A, et al. Comparing cone-beam CT intensity correction methods for dose recalculation in adaptive intensity-modulated photon and proton therapy for head and neck cancer. Acta Oncol 2015;54:1651-7. [Crossref] [PubMed]
- Kurz C, Kamp F, Park YK, et al. Investigating deformable image registration and scatter correction for CBCT-based dose calculation in adaptive IMPT. Med Phys 2016;43:5635. [Crossref] [PubMed]
- Liu W, Frank SJ, Li X, et al. Effectiveness of robust optimization in intensity-modulated proton therapy planning for head and neck cancers. Med Phys 2013;40:051711. [Crossref] [PubMed]
- Han X. Feature-constrained Nonlinear Registration of Lung CT Images. In: MICCAI 2010 Grand Challenges in Medical Image Analysis: Evaluation of Methods for Pulmonary Image Registration (EMPIRE10); 2010:63-72.
- Han X, Hibbard LS, O’Connell NP, et al. Automatic Segmentation of Parotids in Head and Neck CT Images using Multi-atlas Fusion. In: MICCAI: Medical Image Analysis for the Clinic: A Grand Challenge; 2010:297-304.
- Murphy K, van Ginneken B, Reinhardt JM, et al. Evaluation of registration methods on thoracic CT: the EMPIRE10 challenge. IEEE Trans Med Imaging 2011;30:1901-20. [Crossref] [PubMed]
- Han X, Hibbard LS, Willcut V. GPU-accelerated, gradient-free MI deformable registration for atlas-based MR brain image segmentation. In: 2009 IEEE Computer Society Conference on Computer Vision and Pattern Recognition Workshops; 2009:141-8.
- Liu Q, Qin A, Liang J, et al. Evaluation of Atlas-Based Auto-Segmentation and Deformable Propagation of Organs-at-Risk for Head-and-Neck Adaptive Radiotherapy. Recent Pat Top Imaging 2016;5:79-87. [Crossref]
- Liebl J, Paganetti H, Zhu M, et al. The influence of patient positioning uncertainties in proton radiotherapy on proton range and dose distributions. Med Phys 2014;41:091711. [Crossref] [PubMed]
- Thörnqvist S, Muren LP, Bentzen L, et al. Degradation of target coverage due to inter-fraction motion during intensity-modulated proton therapy of prostate and elective targets. Acta Oncol 2013;52:521-7. [Crossref] [PubMed]
- Langius JA, Twisk J, Kampman M, et al. Prediction model to predict critical weight loss in patients with head and neck cancer during (chemo)radiotherapy. Oral Oncol 2016;52:91-6. [Crossref] [PubMed]
- Zhang Z, Zhu Y, Zhang L, et al. Prediction model of critical weight loss in cancer patients during particle therapy. Jpn J Clin Oncol 2018;48:75-81. [Crossref] [PubMed]
- Schatteman J, Van Gestel D, Berwouts D, et al. A feasibility study on adaptive 18F-FDG-PET-guided radiotherapy for recurrent and second primary head and neck cancer in the previously irradiated territory. Strahlenther Onkol 2018;194:727-36. [Crossref] [PubMed]
- Mahmoud O, Reis IM, Samuels MM, et al. Prospective Pilot Study Comparing the Need for Adaptive Radiotherapy in Unresected Bulky Disease and in Postoperative Patients With Head and Neck Cancer. Technol Cancer Res Treat 2017;16:1014-21. [Crossref] [PubMed]
Cite this article as: Wang WG, Qin A, Li X, Zhou J, Yan D, Liu G, Zhao L, Wang Y, Deraniyagala R, Grills I, Stevens C, Krauss D, Ding X. Establish a comprehensive plan robustness evaluation platform for intensity modulated proton therapy for bilateral head and neck cancer based on the daily cone-beam computed tomography: a dose accumulation study between the PTV based IMPT (PTV-IMPT) and CTV based robust optimized IMPT (ro-IMPT) planning strategies. Ther Radiol Oncol 2022;6:9.