Hippocampal avoidance whole brain radiotherapy: developing a RapidPlan model
Introduction
It is estimated that 30% of all cancer patients will develop brain metastases with the rate of this likely to continue to increase with the ongoing improvements in systemic treatments in the palliative setting (1). Recent randomized evidence has now shown the treatment of multiple brain metastases to allow for better quality of life and memory outcomes when patients are given hippocampal-avoidance whole brain radiotherapy (HA-WBRT) with memantine as opposed to WBRT with memantine via simple opposed lateral photons (2,3). Furthermore, HA-WBRT has now been established to provide similar intracranial control and overall survival when utilised for prophylactic cranial irradiation in limited stage small cell lung cancer patients with better neurocognitive preservation as compared with WBRT (4). However, the workflow for this new standard is much more time-consuming, requiring inverse planning often delivered with arc therapy, acquisition and fusion of a diagnostic or simulation MRI, and contouring of additional at-risk structures including the hippocampi.
There is also difficulty in planning these more complex cases, requiring increased planning time due to conflicting objectives, potentially requiring several optimisations with the outcome dependent on the planner’s experience and skills. The requirement that the maximum dose to the hippocampi be approximately 50% of the prescribed dose per Radiation Therapy Oncology Group (RTOG) 0933 constraints (3) is particularly challenging.
To alleviate these challenges, we explore the use of a knowledge-based automated optimisation engine, RapidPlan (Varian Medical Systems, Palo Alto, CA, USA), which is an addition into the Eclipse treatment planning system and aims to achieve improved plan quality and consistency leading to better efficiency in planning (5). The use of RapidPlan has been widely assessed for different anatomical sites (6-13) with this article outlining the creation and testing of an automated HA-WBRT RapidPlan model (Auto). We present the following article in accordance with the GRRAS reporting checklist (available at https://tro.amegroups.com/article/view/10.21037/tro-21-39/rc).
Methods
Contouring and volume generation
Thirty-one consecutive patient datasets in which the whole brain was CT scanned with IV contrast and same-day MRI fusion were identified. Twenty-one patients were selected to create an Auto model and the remaining 10 patients were used to test the model. From these datasets, the hippocampi were contoured by an experienced Radiation Oncologist following the RTOG atlas (14). The hippocampal avoidance structure was formed using an isotropic 5 mm expansion of the volumed hippocampi and this was subtracted from the brain structure to create the planning target volume (PTV). The inferior level of the PTV volume was the inferior border of C1 if there were no posterior fossa metastases or C2 if there was MRI evidence of posterior fossa metastases. The other organs at risk (OARs) contoured include the optic chiasm and optic nerves and in accordance to RTOG guidelines (3).
The PTV was split into two structures, PTV-High (red) and PTV-Low (green), to allow for better optimisation control in the hippocampal region (Figure 1). PTV-Low is defined as the level of the PTV that encompassed the hippocampal region plus 1 slice superior and inferior to this region. This was then subtracted from the PTV to create PTV-High.
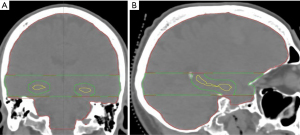
Planning parameters
Prescribed dose was 30 Gy in 10 fractions with PTV coverage requirements in accordance to RTOG 0933 and listed in the table below (Table 1).
Table 1
Site | Coverage | Max | Unacceptable |
---|---|---|---|
PTV 30 | D98% ≥25 Gy (ideal) | D2% ≤37.5 Gy (ideal) | V30 <90% |
D98% <25 Gy (acceptable) | D2% ≤40 Gy (acceptable) | D2% >40 Gy |
PTV, planning target volume.
OAR constraints were as per RTOG 0933 guidelines and listed in the table below (Table 2). The mean doses to lenses and maximum doses to the eyes were also recorded.
Table 2
Structure | Optimal | Acceptable |
---|---|---|
Hippocampus | D100% ≤9 Gy | D100% ≤10 Gy |
Maximum ≤16 Gy | Maximum ≤17 Gy | |
Optic chiasm | Maximum ≤37.5 Gy | – |
Optic nerve | Maximum ≤37.5 Gy | – |
OAR, organ at risk.
Standard template planning
Five arcs were utilised in the planning process with two full arcs at couch 0 degrees and three half arcs at couch 90 with beams eye views in Figure 2 and field details in Table 3. The three half arcs at couch 90 were utilised for optimisation around the hippocampi specifically field 3 for dose optimisation between the hippocampi (Figure 2C), field 4 dose optimisation to the left hippocampi (Figure 2D) and field 5 for dose optimisation to the right hippocampi (Figure 2E). The isocentre was set at the centre of both hippocampi based on the beam’s eye-view. Jaw tracking was also used to reduce the dose to the normal tissue. All plans were optimized with a 6-MV beams on a TrueBeam linac. The manually optimized plans were created for 21 patients and were optimized through the EclipseTM (Varian Medical Systems, Palo Alto, CA, USA) Photon Optimizer v15.6 engine with the final calculations performed with the Anistropic Analytical Algorithm v15.6 at a 2.5 mm dose grid sizes.
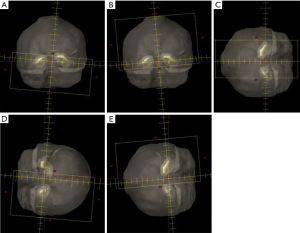
Table 3
Field | Gantry rotation (degrees) | Direction | Collimator rotation (degrees) | Couch rotation (degrees) |
---|---|---|---|---|
1 | 179–181 | CCW | 85 | 0 |
2 | 181–179 | CW | 95 | 0 |
3 | 179–0 | CCW | 90 | 90 |
4 | 0–179 | CW | 85 | 90 |
5 | 179–0 | CCW | 95 | 90 |
Auto, an automated HA-WBRT RapidPlan model; Manual, a manually optimised standard template; CCW, counterclockwise; CW, clockwise.
The order of optimisation priority of the structures was hippocampi as the top priority followed by PTV coverage constraints, lenses and finally the remaining optic apparati. Once all plans achieved the RTOG 0933 PTV coverage requirements and OAR constraints, the plans were assessed by a Radiation Oncologist with the final approved plans for the 21 patients used to create the Auto model. The same standard template planning with manual optimisation approach (Manual) was then applied to the 10 test patients by the same experienced radiation therapist for comparison with RapidPlan produced Auto plans. All plans to create the RapidPlan model as well as all Manual plans were created by the same experienced senior radiation therapist.
Automated RapidPlan model creation
The 21 manually-optimised plans were used to create a v15.6 RapidPlan model by importing 21 Radiation Oncologist approved plans into the dose volume histogram (DVH) estimation model configuration module within Eclipse (Varian Medical Systems, Palo Alto, CA, USA). The software extracts treatment planning information from the imported plans and establishes correlations between plan DVHs, patient anatomy and beam geometry features (15). From the extracted information, the Auto model is trained for each OAR to estimate of DVH curves for new patients.
Automated RapidPlan model validation
This Auto model, without user intervention, was then applied to the 10 test patients to create HA-WBRT plans. For the Auto and Manual plans created on the 10 test patients, DVH data were extracted using the Eclipse Scripting API and compared using the PTV coverage requirements and OAR constraints in Tables 1,2. All plans were normalized to achieve V30 Gy target coverage of 90%. Dose homogeneity was assessed using the homogeneity index (HI), calculated as follows:
A value close to 0 indicates better dose homogeneity within the target volume.
Conformality index (CI) was also calculated as per Paddick et al. (16):
where TV is the target volume, TVPTV is the target volume covered by the prescription isodose, and VRI is the total volume covered by the prescription isodose with a CI close to 1 indicating better dose conformity to the target volume size and shape.
Patient specific quality assurance measurements were then performed on the 10 Auto plans to ensure accuracy and deliverability.
Statistical analysis
Statistical comparisons and graphs of the results were performed using MatlabTM with an unpaired two-sample t-test used where P<0.05 indicates significance in the difference of the mean values. Boxplots were produced to compare results. These display the interquartile range as a blue box with the median indicated by a red line and outliers shown as red ‘+’ symbols.
Ethical statement
The study was conducted in accordance with the Declaration of Helsinki (as revised in 2013). The study was approved by the Sydney Local Health District-Royal Prince Alfred Ethics Review Committee (reference: X20-0304 & 2020/ETH01446). Consent from patients was not required as datasets and plans were not for clinical use for these patients nor did they affect their management and solely for the creation and validation of the department-specific RapidPlan model.
Results
Both the Auto and Manual approach were able to produce plans that achieved protocol requirements. However, the Auto approach was able to achieve these requirements in its 1st optimisation for all 10 patients whereas the Manual was only able to produce acceptable plans in a single optimisation for 5 patients, with 4 patients requiring 2 optimisations and 1 patient requiring 3 optimisations to achieve protocol requirements. Regarding the Manual plan which required 3 optimisations, the main conflict was in balancing mean lens dose with hippocampi dose constraints.
The PTV coverages for the Auto plans were slightly lower than for the Manual plans but all plans easily achieved the ideal coverage level (Figure 3), with D98% greater than 25 Gy for all plans and V30 Gy coverage 90.2% for Manual plans, V30 Gy coverage 90.0% for Auto plans (P=0.405).
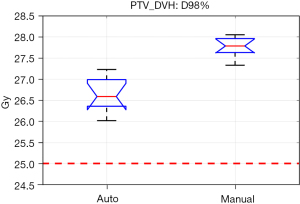
Importantly, the Auto plans achieved significantly lower hippocampal doses, with mean D100% for the right hippocampus at 8.51 Gy for and 9.22 Gy for the Manual plans (P<0.001) (Figure 4). Mean D100% for the left hippocampus was 8.56 Gy for the Auto planning and 9.24 Gy for the Manual plans with only the Auto plans meeting the ideal RTOG constraint of D100% ≤9 Gy for both hippocampi. The maximum hippocampal dose was also significantly better with Auto planning, at 14.1 and 16.2 Gy respectively for Auto and Manual for the right, and 14.0 and 16.1 Gy for the left (P<0.001) with Auto plans of the two meeting ideal maximal dose constraints for both hippocampi.
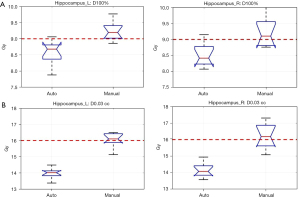
As an example, Figure 5 is a dose colour wash distribution showing the 16 Gy isodose wrapping around the hippocampi for the Auto plan whereas the 16 Gy isodose is within the hippocampal region for the Manual plan.
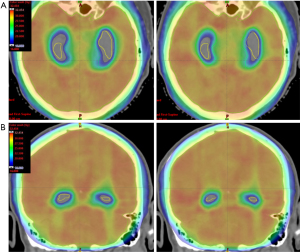
In terms of the other OARs, maximum dose was less than 37.5 Gy for the optic chiasm and optic nerves for all plans, meeting the optimal dose constraints for RTOG 0933 in both Auto and Manual plans. A mean lens dose of less than 6 Gy was achieved for all Auto plans while the Manual planning failed to achieve this for 1 patient.
In assessing HI and CI between the Auto and Manual plans, the values were all comparable to each other with low HI and high CI (Table 4). The monitor units and complexity of the auto and manual plans were compared using a complexity analysis (17). The Auto plans were found to be more complex (P=0.02) as would be expected. However, as the fluences were simple in both plan sets this did not lead to reduced deliverability. The Auto plans had higher monitor units but this was not significant (P=0.76).
Table 4
Auto | Manual | ||||
---|---|---|---|---|---|
HI | CI | HI | CI | ||
Mean | 0.16 | 0.83 | 0.13 | 0.83 | |
SD | 0.02 | 0.02 | 0.01 | 0.01 |
HI, homogeneity index; CI, conformality index; Auto, an automated HA-WBRT RapidPlan model; Manual, a manually optimised standard template; SD, standard deviation.
An independent dose calculation and patient specific dosimetry measurement were performed for all 10 Auto plans with all easily achieving the required tolerance levels. The independent dose calculation was performed using SunCHECKTM with point doses for each field agreeing within 3% and PTV gamma pass rates greater than 99% with a 3%/2 mm tolerance.
Patient specific dosimetry measurements were performed using an ArcCheckTM device with pass rates using a 3%/2 mm gamma assessment of 98.8% or above for all fields. In addition, Portal DosimetryTM measurements were performed and all arc fields passed a 3%/3 mm tolerance at greater than 99% in relative mode. Based on the high pass rates observed and the consistency of the plans produced, ongoing patient specific quality assurance has been streamlined to include only an independent dose check with SunCheck and a Portal Dosimetry fluence check.
Discussion
The Phase III NRG CC001 randomised trial results recently confirmed HA-WBRT with memantine to be superior to WBRT with memantine in diffuse cognitive parameters as well as quality of life with no difference in overall or intracranial progression free survival (2). This new standard of care treatment for multiple brain metastases is of great importance as neurocognitive toxicity has been a major contributor in the diminishing use of traditional WBRT. The PREMER randomized study this year also provided evidence for neurocognitive preservation in prophylactic cranial irradiation with hippocampal avoidance in small cell lung cancer (4), further increasing the utility of HA-WBRT. However, workflow for HA-WBRT is significantly more complicated, requiring up-to-date MRI brain acquisition and fusion, OAR contouring, and complex planning.
To streamline workflow, our prior study assessed whether auto-contouring of the hippocampi via Elements Treatment Planning System had reasonable conformality to clinician manual contours using the RTOG atlas (18). This paper outlines the creation of a department-specific HA-WBRT Auto RapidPlan model and its validation using RTOG 0933 dosimetric parameters and Radiation Oncologist final approval to allow for further downstream efficiency.
Our results first showed that creation of an Auto model from our manually optimised plans using 21 patient plans is feasible. Historically, the number of cases required to create an automated RapidPlan model varied, ranging from 20 to almost 200 (11,19-24), with number of cases likely at least partially dependent on the subsite being assessed. Ueda et al. (20) suggested that only 20 cases might be enough to create a model if large variations existed in the registered cases. This was evident in our study, with our Auto model containing 21 patients able to achieve a single optimisation plan meeting all plan requirements for the 10 study patients. Similarly, Rusu et al. (19) utilised an outside-sourced RapidPlan model for HA-WBRT created with 20 patients and were able to produce plans which achieved RTOG 0933 requirements in a single optimization. The fewer cases required is at least partly due to the brain being a relatively stable structure with less variation in anatomy than other sites and the standardized beam arrangement used. This allows for a more expedited process for creation of a department-specific automated RapidPlan model.
A point of difference with Rusu et al. (19) was their utilisation of an outside RapidPlan model which they then validated. Our group built our own model which can be invaluable in creating a local model as it considers local planning practices, included contouring, treatment technique and planning goals (25). Schubert et al. (26) explored model sharing amongst multiple centres finding that although all cases of RapidPlan plans were clinically acceptable some OARs were better spared in some centres compared to others. This was due to different contouring protocols or emphasis in structures for optimisation priority and certainly we have previously interrogated adherence of our in-house hippocampi contouring to the RTOG atlas (18). Additionally, Ueda et al. (20) suggest that for models to be shared successfully that plan design should match between institutions as values created with RapidPlan are influenced by plans contained in the model. A further point of consideration include departmental machine capabilities which may not be considered by an external model.
Our automated RapidPlan model was validated on 10 further patients in comparison with radiation therapist-created manual standard template minimal optimisations required for plan generation that met or exceeded RTOG 0933 plan requirements, thus reducing time and staffing demands for plan creation. Auto plans also had significantly lower hippocampal doses, optimizing neurocognitive preservation with this memory-critical structure. Doses to optic nerves, chiasm and lenses were also lower with Auto plans, again potentially further reducing toxicity. The results did show a slightly lower dose to the PTV D98 in the Auto plans compared to the Manual plans and this was because our top priority was to minimise the dose to the hippocampi while still meeting optimal dose coverage requirements, the goal of which was met.
The dosimetric verification of the plans prior to implementation of the model clinically demonstrates that the plans created are deliverable but also reduces the chance of patient delays due to failing dosimetric measurements. The simplification of patient specific quality assurance based on a standardized and reproducible planning method reduces the physics resource requirements and expedites the physics checking process contributing to a streamlined workflow and efficiency of clinical implementation.
Conclusions
This paper has shown the feasibility of an automated HA-WBRT RapidPlan model created from 21 standard template plans with department-specific prioritizations. The department specific automated RapidPlan model was then validated in comparison with plans optimized by a standard template of objectives with plans generated meeting RTOG 0933 constraints on first optimization and with significantly better hippocampal doses as well as lower optic apparati and lens doses. The plans were also all dosimetrically verified for clinical implementation, mimicking clinical practice and ensuring deliverability. This paves the way for true clinical implementation and real world streamlining of HA-WBRT treatment with quicker plan turnaround and minimized resource utilisation.
Acknowledgments
This study was conducted at the Chris O’Brien Lifehouse, Camperdown, Australia.
Funding: None.
Footnote
Reporting Checklist: The authors have completed the GRRAS reporting checklist. Available at https://tro.amegroups.com/article/view/10.21037/tro-21-39/rc
Conflicts of Interest: All authors have completed the ICMJE uniform disclosure form (available at https://tro.amegroups.com/article/view/10.21037/tro-21-39/coif). The authors have no conflicts of interest to declare.
Ethical Statement: The authors are accountable for all aspects of the work in ensuring that questions related to the accuracy or integrity of any part of the work are appropriately investigated and resolved. The study was conducted in accordance with the Declaration of Helsinki (as revised in 2013). The study was approved by the Sydney Local Health District-Royal Prince Alfred Ethics Review Committee (reference: X20-0304 & 2020/ETH01446). Consent from patients was not required as datasets and plans were not for clinical use for these patients nor did they affect their management and solely for the creation and validation of the department-specific RapidPlan model.
Open Access Statement: This is an Open Access article distributed in accordance with the Creative Commons Attribution-NonCommercial-NoDerivs 4.0 International License (CC BY-NC-ND 4.0), which permits the non-commercial replication and distribution of the article with the strict proviso that no changes or edits are made and the original work is properly cited (including links to both the formal publication through the relevant DOI and the license). See: https://creativecommons.org/licenses/by-nc-nd/4.0/.
References
- Brown PD, Ahluwalia MS, Khan OH, et al. Whole-Brain Radiotherapy for Brain Metastases: Evolution or Revolution? J Clin Oncol 2018;36:483-91. [Crossref] [PubMed]
- Brown PD, Gondi V, Pugh S, et al. Hippocampal Avoidance During Whole-Brain Radiotherapy Plus Memantine for Patients With Brain Metastases: Phase III Trial NRG Oncology CC001. J Clin Oncol 2020;38:1019-29. [Crossref] [PubMed]
- Gondi V, Pugh SL, Tome WA, et al. Preservation of memory with conformal avoidance of the hippocampal neural stem-cell compartment during whole-brain radiotherapy for brain metastases (RTOG 0933): a phase II multi-institutional trial. J Clin Oncol 2014;32:3810-6. [Crossref] [PubMed]
- Rodríguez de Dios N, Couñago F, Murcia-Mejía M, et al. Randomized Phase III Trial of Prophylactic Cranial Irradiation With or Without Hippocampal Avoidance for Small-Cell Lung Cancer (PREMER): A GICOR-GOECP-SEOR Study. J Clin Oncol 2021;39:3118-27. [Crossref] [PubMed]
- van Gysen K, O'Toole J, Le A, et al. Rolling out RapidPlan: What we've learnt. J Med Radiat Sci 2020;67:310-7. [Crossref] [PubMed]
- Fogliata A, Wang PM, Belosi F, et al. Assessment of a model based optimization engine for volumetric modulated arc therapy for patients with advanced hepatocellular cancer. Radiat Oncol 2014;9:236. [Crossref] [PubMed]
- Hussein M, South CP, Barry MA, et al. Clinical validation and benchmarking of knowledge-based IMRT and VMAT treatment planning in pelvic anatomy. Radiother Oncol 2016;120:473-9. [Crossref] [PubMed]
- Fogliata A, Nicolini G, Clivio A, et al. A broad scope knowledge based model for optimization of VMAT in esophageal cancer: validation and assessment of plan quality among different treatment centers. Radiat Oncol 2015;10:220. [Crossref] [PubMed]
- Foy JJ, Marsh R, Ten Haken RK, et al. An analysis of knowledge-based planning for stereotactic body radiation therapy of the spine. Pract Radiat Oncol 2017;7:e355-60. [Crossref] [PubMed]
- Tol JP, Delaney AR, Dahele M, et al. Evaluation of a knowledge-based planning solution for head and neck cancer. Int J Radiat Oncol Biol Phys 2015;91:612-20. [Crossref] [PubMed]
- Fogliata A, Reggiori G, Stravato A, et al. RapidPlan head and neck model: the objectives and possible clinical benefit. Radiat Oncol 2017;12:73. [Crossref] [PubMed]
- Fogliata A, Nicolini G, Bourgier C, et al. Performance of a Knowledge-Based Model for Optimization of Volumetric Modulated Arc Therapy Plans for Single and Bilateral Breast Irradiation. PLoS One 2015;10:e0145137. [Crossref] [PubMed]
- Chin Snyder K, Kim J, Reding A, et al. Development and evaluation of a clinical model for lung cancer patients using stereotactic body radiotherapy (SBRT) within a knowledge-based algorithm for treatment planning. J Appl Clin Med Phys 2016;17:263-75. [Crossref] [PubMed]
- Gondi V, Tolakanahalli R, Mehta MP, et al. Hippocampal-sparing whole-brain radiotherapy: a "how-to" technique using helical tomotherapy and linear accelerator-based intensity-modulated radiotherapy. Int J Radiat Oncol Biol Phys 2010;78:1244-52. [Crossref] [PubMed]
- Elcipse Photon and Electron Algorithms Reference Guide. Palo Alto: Varian Medeical Systems, Inc., 2018: P1008611-003-C.
- Paddick I. A simple scoring ratio to index the conformity of radiosurgical treatment plans. Technical note. J Neurosurg 2000;93:219-22. [Crossref] [PubMed]
- Younge KC, Roberts D, Janes LA, et al. Predicting deliverability of volumetric-modulated arc therapy (VMAT) plans using aperture complexity analysis. J Appl Clin Med Phys 2016;17:124-31. [Crossref] [PubMed]
- Lo H, Estoesta P, Mackonis EC, et al. Clinical evaluation of RTOG-atlas based contouring, MRI-atlas auto-contouring, and neuroradiologist contouring of the hippocampi for hippocampal avoidance whole-brain radiotherapy. Ther Radiol Oncol 2021;5:19. [Crossref]
- Rusu I, Roeske J, Solanki A, et al. Fully automated planning and delivery of hippocampal-sparing whole brain irradiation. Med Dosim 2022;47:8-13. [Crossref] [PubMed]
- Ueda Y, Fukunaga JI, Kamima T, et al. Evaluation of multiple institutions' models for knowledge-based planning of volumetric modulated arc therapy (VMAT) for prostate cancer. Radiat Oncol 2018;13:46. [Crossref] [PubMed]
- Panettieri V, Ball D, Chapman A, et al. Development of a multicentre automated model to reduce planning variability in radiotherapy of prostate cancer. Phys Imaging Radiat Oncol 2019;11:34-40. [Crossref] [PubMed]
- Castriconi R, Esposito PG, Tudda A, et al. Replacing Manual Planning of Whole Breast Irradiation With Knowledge-Based Automatic Optimization by Virtual Tangential-Fields Arc Therapy. Front Oncol 2021;11:712423. [Crossref] [PubMed]
- Harms J, Zhang J, Kayode O, et al. Implementation of a Knowledge-Based Treatment Planning Model for Cardiac-Sparing Lung Radiation Therapy. Adv Radiat Oncol 2021;6:100745. [Crossref] [PubMed]
- Wu H, Jiang F, Yue H, et al. Applying a RapidPlan model trained on a technique and orientation to another: a feasibility and dosimetric evaluation. Radiat Oncol 2016;11:108. [Crossref] [PubMed]
-
Mackonis ERC Sykes J Hardcastle N A Comparison of in-house and Shared Rapidplan Models for Prostate Radiation Therapy Planning. Phys Eng Sci Med 2022 . In Press. doi: .10.21203/rs.3.rs-958580/v1 - Schubert C, Waletzko O, Weiss C, et al. Intercenter validation of a knowledge based model for automated planning of volumetric modulated arc therapy for prostate cancer. The experience of the German RapidPlan Consortium. PLoS One 2017;12:e0178034. [Crossref] [PubMed]
Cite this article as: Lo H, Estoesta P, Mackonis EC, O’Connor M, Choong ES. Hippocampal avoidance whole brain radiotherapy: developing a RapidPlan model. Ther Radiol Oncol 2022;6:13.