Hippocampus-sparing whole-brain radiotherapy: dosimetric comparison between non-coplanar and coplanar planning
Introduction
Brain metastases are the most common intracranial tumors in adults and occur in 10–30% of cancer patients (1). The prognosis of patients with brain metastases is generally poor, with a median survival time of one month in untreated patients and 4–6 months in treated patients (2). Many cancer patients will eventually develop brain metastases, and the standard therapeutic approaches for patients with brain metastasis include surgery and radiotherapy. Whole-brain radiotherapy (WBRT) has been implemented in clinical practice for decades. In general, patients with oligo-brain metastasis undergo surgical resection, chemotherapy and stereotactic radiosurgery (SRS) alone or SRS combined with WBRT (3). For patients with multiple brain metastases, WBRT is a commonly used treatment modality; however, SRS seems to be a new standard option (4).
WBRT plays an important role in tumor control for patients with multiple brain tumors and thus lower the subsequent neurological death. WBRT is also used in prophylactic cranial irradiation for patients with small cell lung carcinoma (5-7). Although WBRT was proven to be effective in eliminating brain tumors, damage to normal brain tissue is inevitable, and several complications have been observed following treatment that decreases the quality of life of patients (8). Previous studies showed that poor neurocognitive function may occur months after radiotherapy due to radiation-induced hippocampal damage. The radiation-induced hippocampal damage causes a decline in neurocognitive function, including deficiencies in learning, memory and spatial management, which can be observed in patients. The clinical symptoms of radiation-induced hippocampal damage include memory loss, lack of concentration, and emotional disorders (9,10).
The findings of radiation therapy oncology group (RTOG) 0933 trial suggest that hippocampal avoidance during WBRT (HA-WBRT) could reduce radiation-induced neurocognitive toxicities (11). The purpose of HA-WBRT is to generate hippocampal avoidance regions while allowing the sufficient prescribed dose (PD) to cover the remainder of the brain. HA-WBRT can significantly reduce the hippocampal dose. Although the risk of developing subsequent brain metastasis after HA-WBRT is unknown, Ghia et al. (12) reported that of 272 cases with brain metastases, the incidence of metastases within 5 mm of the perihippocampal region was low (3.3%). Thus, their results indicated that a 5-mm margin around the hippocampus for HA-WBRT was an acceptable risk. With advances in radiotherapy, several reports have discussed the application of intensity-modulated radiotherapy (IMRT) and volumetric-modulated arc therapy (VMAT) for HA-WBRT and attempted to minimize WBRT-induced neurocognitive toxicities. Nevelsky et al. (13) evaluated the feasibility of the RTOG 0933 trial using the Elekta Infinity (Elekta AB, Stockholm, Sweden) equipped with Monaco 3.1 (Elekta AB, Stockholm, Sweden) treatment planning system (TPS) to perform IMRT with seven coplanar plus two non-coplanar beams. VMAT, with continuous gantry rotation and leaf motion with a varying dose rate, can increase treatment efficiency and reduce the amount of patient motion during the treatment (14). Therefore, the purpose of this study is to compare the non-coplanar and coplanar VMAT for HA-WBRT using the Elekta Synergy (Elekta AB) and Pinnacle (Philips Medical System, Fitchburg, WI, USA) TPS according to the suggested criteria of the RTOG 0933 trial. We also performed VMAT quality assurance (QA) for each treatment plan to evaluate the feasibility of HA-WBRT with different VMAT techniques.
We present the following article in accordance with the MDAR reporting checklist (available at http://dx.doi.org/10.21037/tro-20-50).
Methods
Patient selection and delineation
The study was approved by the Taipei Medical University Joint Institutional Review Board, and the IRB number is TMU-JIRB No. N201711047. Nine patients with brain metastases outside 5 mm of the hippocampus who underwent WBRT were selected for this retrospective study. Computed tomography (CT) and magnetic resonance (MR) images were used for each patient to delineate the hippocampus, hippocampal avoidance regions (defined as the hippocampus with an expansion of 5-mm margin) according to the RTOG 0933 trial, optic nerves, optic chiasm, and lens. CT images (3 mm slice thickness) for treatment planning were generated using a Phillips 16 slice CT scanner (Brilliance CT Big Bore). All 6-MV VMAT plans were optimized with Pinnacle3 Version 14 for Elekta Synergy with 40 multileaf collimator (MLC) leaf pairs (MLCi) of 1 cm leaf width at isocenter.
The study was conducted in accordance with the Declaration of Helsinki (as revised in 2013). The study was approved by the Taipei Medical University Joint Institutional Review Board (TMU-JIRB No. N201711047), and informed consent was taken from all the patients.
Planning technique
According to the RTOG 0933 trial, the clinical target volume (CTV) was defined as the whole brain parenchyma to C1, and the planning treatment volume (PTV) was defined as the CTV excluding the hippocampal avoidance regions in this study. A total dose of 30 Gy in 10 fractions was prescribed to the PTV. All plans in this study aimed to cover at least 90% of the PTV by the PD. The following dose constraints, which are summarized in Table 1, were used in optimization: maximum dose to 2% of the PTV (PTV D2%) ≤37.5 Gy; minimum dose to 98% of the PTV (PTV D98%) ≥25 Gy; D100% (Dx%: the dose to X% of the volume) and maximum dose (Dmax) of the hippocampus ≤9 Gy and ≤16 Gy; Dmax of the optic chasm and optic nerve <37.5 Gy; and doses to the lens and optic nerve as low as possible. The non-coplanar and coplanar VMAT plans were generated for each patient. For the coplanar VMAT plans, four full arcs (FAs) (clockwise for two FAs and counterclockwise for two FAs) were used with a collimator angle of 45°. For the non-coplanar VMAT plans, five arcs, including one partial arc (PA) with a collimator angle of 45° and couch angle of 70° and four FAs (clockwise for two FAs and counterclockwise for two FAs), were used (Table 2).
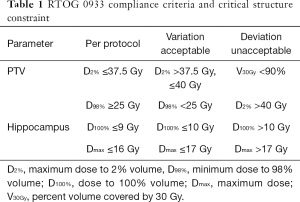
Full table
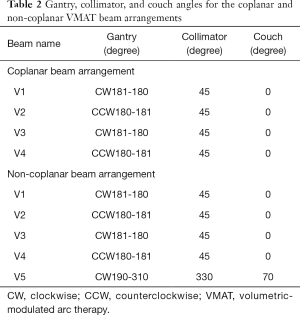
Full table
Plan evaluation
For the organs at risk (OARs) and PTV, the following parameters were evaluated: PTV D2%, PTV D98%, D100% and Dmax of the hippocampus, and Dmax of the optic chiasm and lens. The dosimetric parameters, including PTV V100 (PTV VX is the percent volume of PTV covered by X% of the PD), PTV V95, homogeneity index (HI) and conformity index (CI) for the PTV, were evaluated.
The HI is defined as follows (recommended by the ICRU 83) (15):
where Dmedian is the median dose of the PTV.
The CI is defined as follows (16):
where VPTV is the volume of PTV, and V95 is the volume covered by 95% of the PD.
The beam-on time was defined as the treatment time excluding the time for patient setup and couch rotation. The beam-on times for different VMAT plans were reported and evaluated. The VMAT QA procedures were completed for each plan and performed by measuring the dose distribution using the PTV 2D-array seven29 with a plane matrix of 27×27 air-filled ion chambers (17).
Statistical analysis
The Wilcoxon signed-rank test was used for statistical analysis among different VMAT techniques. A P value of ≤0.05 was considered statistically significant.
Results
The basic characteristics of the nine patients are listed in Table 3. The average volume of the hippocampus was 2.80 cm3 (range, 2.32–3.40 cm3), the average volume of the hippocampal avoidance region was 27.09 cm3 (range, 24.76–31.26 cm3), and the average volume of the whole brain was 1,314.35 cm3 (range, 1,116.01–1,479.70 cm3).
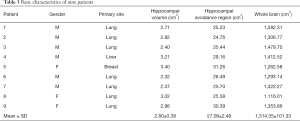
Full table
In all treatment plans, the dose constraints for the hippocampus according to the RTOG 0933 trial were prioritized while limiting the dose to the lens. Figure 1 shows the isodose lines (ranging from 1,500 to 3,400 cGy) around the hippocampus of the non-coplanar and coplanar VMAT plans on axial, coronal, and sagittal images from one case of this study. The average DVHs of the hippocampus, lens and PTV for the non-coplanar and coplanar VMAT plans for nine patients are shown in Figure 2.
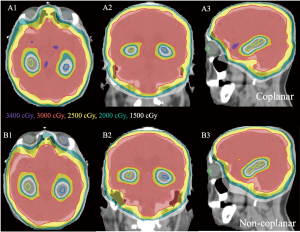
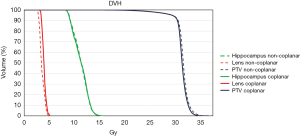
Dose to OARs
Table 4 shows the dose to the OARs for the non-coplanar and coplanar VMAT plans. The non-coplanar VMAT plans had a lower mean Dmax of the hippocampus than the coplanar VMAT plans, but the difference was nonsignificant (P>0.05): coplanar VMAT =15.29 Gy (range, 14.35–15.92 Gy) and non-coplanar VMAT =14.99 Gy (range, 13.80–15.83 Gy). The average D100% values of the hippocampus for the coplanar and non-coplanar VMAT plans were 8.60 Gy (range, 8.30–8.80 Gy) and 8.56 Gy (range, 8.30–8.90 Gy), respectively. The non-coplanar VMAT plans showed a significantly lower average Dmax of the lens (P<0.05) than the coplanar VMAT plans: coplanar VMAT =4.77 Gy (range, 3.99–5.50 Gy) and non-coplanar VMAT =4.23 Gy (range, 3.65–5.07 Gy). The dose to the optic chiasm for all plans did not exceed 37.5 Gy.
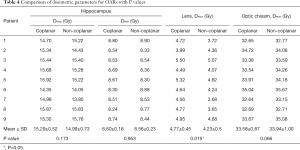
Full table
PTV dosimetry
The PTV D2%, PTV D98%, PTV V30Gy (the percent volume of PTV covered by 30 Gy), PTV V95, target coverage, HI and CI are shown in Table 5. The average PTV D2%, PTV D98%, and PTV V30Gy for the non-coplanar and coplanar VMAT plans were 33.46±0.68 and 33.19±0.47 Gy, 25.49±0.48 and 25.68±0.63 Gy, and 92.99%±0.35% and 92.57%±0.80%, respectively.
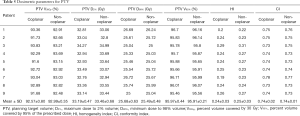
Full table
Beam-on time and VMAT QA
The beam-on time and VMAT QA results for nine patients are shown in Table 6. There was no significant difference (P>0.05) in the beam-on time between the non-coplanar (average, 6.2±1.3 min) and coplanar VMAT plans (average, 5.6±0.5 min). The results of the VMAT QA are shown in Figure 3. The average gamma passing rates for the non-coplanar and coplanar VMAT QA with criteria of 3%/3 mm were 95.4%±2.6% and 95.6%±1.6%, respectively, which indicated good agreement between the calculated plan dose and the measured dose.
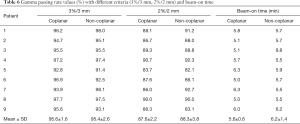
Full table
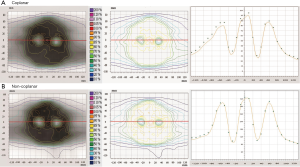
Discussion
Nevelsky et al. (13) evaluated the feasibility of the RTOG 0933 trial using the Elekta Infinity to perform IMRT with seven coplanar plus two non-coplanar beams. We hypothesized that VMAT could achieve the same results. In this study, we performed HA-WBRT using non-coplanar and coplanar VMAT with Elekta Synergy. Our results showed that for all VMAT plans, the hippocampal dose can achieve the suggested criteria of the RTOG 0933 trial, with Dmax of the PTV (PTV Dmax) lower than 37.5 Gy, while maintaining the PTV coverage (at least 90% of the PTV by the PD).
In comparison with the study of Nevelsky et al. (13), their results showed that the average PTV D98% was 25.7 Gy, PTV D2% was 37.2 Gy, HI was 0.36, and Dmax of the hippocampus was 14.3 Gy, and our results showed that the average Dmax of the hippocampus was slightly higher (14.9 Gy); however, our results showed better average PTV D2% (33.46 Gy), PTV D98% (25.49 Gy), and HI (0.25).
Gondi et al. (18) proposed 9-beam non-planar IMRT with nine different couch angles for HA-WBRT, and their results showed that the average Dmax of the hippocampus was 15.3 Gy, and the average HI was 0.3. Our non-coplanar VMAT plans showed better average Dmax and HI values than those of Gondi et al. (18), and the shorter treatment time for the non-coplanar VMAT of this study can be expected due to less couch angle adjustment (0° and 70°).
Krayenbuehl et al. (19) evaluated the feasibility of HA-WBRT according to the RTOG 0933 trial using an automated TPS with VMAT of two FAs and two non-coplanar PAs. Their results showed that for 10 patients, the average Dmax and minimum dose (Dmin) of the hippocampus were 14.1 Gy (range, 12–15.3 Gy) and 8.1 Gy (range, 7.8–8.5 Gy), respectively; the average PTV V30Gy and PTV Dmax were 92% and 36 Gy (range, 35.1–36.5 Gy), respectively. In this study, we used the same TPS as the study performed by Krayenbuehl et al. (19), and our results for the non-coplanar VMAT plans showed that the average Dmax and Dmin of the hippocampus were 14.9 Gy (range, 13.8–15.8 Gy) and 8.5 Gy (range, 8.3–8.8 Gy). For the PTV, Krayenbuehl et al. (19) aimed to reduce the high dose in the normal brain (i.e., PTV D2%), and the average PTV D2% of their study was 33.5 Gy. In our study, the average PTV D2% was 33.4 Gy. Although our study showed a higher average PTV coverage (92.9%) than that reported by Krayenbuehl et al. (19) (92.0%), the Dmax and Dmin of the hippocampus in our study were both higher than their results but still met the criteria of the RTOG 0933 trial. Therefore, our results indicated that this study could reduce the high dose in the brain while maintaining PTV coverage. As for the better Dmax and Dmin of the hippocampus in the study of Krayenbuehl et al. (19), we believe that this can be explained by the usage of a Trilogy linac (Varian Medical System, Palo Alto, CA, USA) with 60 MLC pairs of 0.5 cm leaf width at isocenter. The narrower MLCs of the Trilogy compared with the MLCs of the Elekta Synergy used in this study can affect the treatment plan quality, since the width of the MLC would affect the spatial resolution of the dose distribution, and the penumbra width of the MLC could determine the dose fall-off in the hippocampal avoidance regions (20).
In the study performed by Wang et al. (21) using coplanar VMAT with two FAs, the average PTV D2% and PTV D98% were 35.1 and 26.6 Gy, respectively, and the Dmax and Dmin of the hippocampus were 16 and 9.3 Gy, respectively. In this study, four FAs were used for the coplanar VMAT, which allowed us to achieve better PTV D2%, PTV D98%, and Dmax and Dmin values of the hippocampus than those reported by Wang et al. (21). Based on the results, we speculate that increasing the arc number of VMAT could be used to easily achieve the dose constraints of the RTOG 0933 trial but would increase the treatment time as well. The dosimetric comparison data from the studies mentioned above and our study are summarized in Table 7.
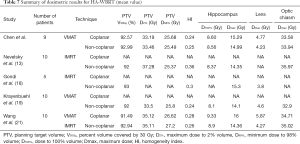
Full table
For Dmax and Dmin of the hippocampus, our results indicated that no significant differences were observed between the non-coplanar and coplanar VMAT plans. For Dmax of the lens, the non-coplanar VMAT plans showed a significantly lower value than the coplanar VMAT plans. Previously reported of radiation-induced lens change in animal and human radiation cataract in radiation workers, exposure of the lens to ionizing radiation can result in opacification (22). Nguyen et al. (23) proposed a mean lens dose threshold of 7 Gy, and they reported that the cataract risk can be reduced to less than 25%. In addition, the National Council on Radiation Protection and Measurements No. 26 report suggests that it is more advantageous to keep the lens doses as low as possible according to the as low as reasonably achievable principle (24).
The non-coplanar technique could decrease the Dmax of the lens; however, due to the couch rotation during the treatment, the rotation accuracy for couch and gantry needs to be assessed before the non-coplanar treatment. The difference in treatment managements in clinical practice between the coplanar and non-coplanar VMAT plans results in the difference in delivery time. Although there was no statistically significant difference in the beam-on time between the non-coplanar (average, 6.2 min) and coplanar VMAT plans (average, 5.6 min). In clinical practice, however, coplanar VMAT is performed with a fixed couch angle, and the couch angle needs to be adjusted for the non-coplanar VMAT. Therefore, we believe that the coplanar VMAT is less time-consuming than the non-coplanar VMAT. The shorter treatment time can reduce patient discomfort and decrease patient irritability during treatment.
Because VMAT involves continuous gantry rotation and leaf motion with a varying dose rate, it is important to verify the consistency of the TPS and beam delivery. In this study, we performed VMAT QA for each treatment plan to evaluate the feasibility of HA-WBRT. On average, the gamma passing rate with criteria of 3%/3 mm was above 90%, within the action level of 90% recommended by the AAPM Task Group 119 report (25). The profile shown in Figure 3 demonstrates that for the hippocampus, the measured dose distribution was in good agreement with the calculated plan dose distribution.
Conclusions
The application of VMAT for HA-WBRT has been a trend in clinical treatment for patients with brain metastases, and one advantage is the significant reduction in treatment time. This study shows that the suggested criteria of the RTOG guidelines for the hippocampal dose can be achieved in both non-coplanar and coplanar VMAT plans. The non-coplanar VMAT plans showed a significantly lower Dmax of the lens than the coplanar VMAT plans. However, for the doses to other OARs, the coplanar VMAT plans showed comparable results. In addition, better treatment efficiency for coplanar VMAT than non-coplanar VMAT can be expected in clinical practice due to the shorter beam-on time and fixed couch angle. This study provides a dosimetric comparison of two different VMAT techniques for HA-WBRT, and we performed VMAT QA for each treatment plan to verify the clinical feasibility.
Acknowledgments
Funding: The authors disclosed receipt of the following financial support for the research and publication of this article: this work was supported by Taipei Medical University - Shuang-Ho Hospital (grant number: 107HCP-11).
Footnote
Reporting Checklist: The authors have completed the MDAR reporting checklist. Available at http://dx.doi.org/10.21037/tro-20-50
Conflicts of Interest: All authors have completed the ICMJE uniform disclosure form (available at http://dx.doi.org/10.21037/tro-20-50). All authors report grants from Taipei Medical University - Shuang-Ho Hospital, outside the submitted work.
Ethical Statement: The authors are accountable for all aspects of the work in ensuring that questions related to the accuracy or integrity of any part of the work are appropriately investigated and resolved. The study was conducted in accordance with the Declaration of Helsinki (as revised in 2013). The study was approved by the Taipei Medical University Joint Institutional Review Board (TMU-JIRB No. N201711047), and informed consent was taken from all the patients
Open Access Statement: This is an Open Access article distributed in accordance with the Creative Commons Attribution-NonCommercial-NoDerivs 4.0 International License (CC BY-NC-ND 4.0), which permits the non-commercial replication and distribution of the article with the strict proviso that no changes or edits are made and the original work is properly cited (including links to both the formal publication through the relevant DOI and the license). See: https://creativecommons.org/licenses/by-nc-nd/4.0/.
References
- Khuntia D, Brown P, Li J, et al. Whole-brain radiotherapy in the management of brain metastasis. J Clin Oncol 2006;24:1295-304. [Crossref] [PubMed]
- Li J, Bentzen SM, Li J, et al. Relationship between neurocognitive function and quality of life after whole-brain radiotherapy in patients with brain metastasis. Int J Radiat Oncol Biol Phys 2008;71:64-70. [Crossref] [PubMed]
- Chiesa S, Balducci M, Azario L, et al. Development of a modelling to correlate site and diameter of brain metastases with hippocampal sparing using volumetric modulated Arc therapy. Biomed Res Int 2013;2013:568597. [Crossref] [PubMed]
- Kraft J, Zindler J, Minniti G, et al. Stereotactic Radiosurgery for Multiple Brain Metastases. Curr Treat Options Neurol 2019;21:6. [Crossref] [PubMed]
- Sood S, Pokhrel D, McClinton C, et al. Volumetric-modulated arc therapy (VMAT) for whole brain radiotherapy: not only for hippocampal sparing, but also for reduction of dose to organs at risk. Med Dosim 2017;42:375-83. [Crossref] [PubMed]
- Prado A, Milanés AI, Cabello E, et al. Dosimetric comparison of four volumetric-modulated arc therapy beam arrangements utilized for hippocampal-sparing whole-brain radiation therapy. J Med Phys 2019;44:1-8. [Crossref] [PubMed]
- Rong Y, Evans J, Xu-Welliver M, et al. Dosimetric evaluation of intensity-modulated radiotherapy, volumetric modulated arc therapy, and helical tomotherapy for hippocampal-avoidance whole brain radiotherapy. PLoS One 2015;10:e0126222. [Crossref] [PubMed]
- Warrington JP, Ashpole N, Csiszar A, et al. Whole brain radiation-induced vascular cognitive impairment: mechanisms and implications. J Vasc Res 2013;50:445-57. [Crossref] [PubMed]
- Aoyama H, Shirato H, Tago M, et al. Stereotactic radiosurgery plus whole-brain radiation therapy vs stereotactic radiosurgery alone for treatment of brain metastases: a randomized controlled trial. JAMA 2006;295:2483-91. [Crossref] [PubMed]
- De Felice F, Musio D, Cassese R, et al. Radiotherapeutic treatment approaches for brain metastases. Anticancer Res 2014;34:6913-8. [PubMed]
- Mehta MP, Gondi V, Corn B, et al. A phase II trial of hippocampal avoidance during whole brain radiotherapy for brain metastases. RTOG 0933. Philadelphia, Pennsylvania: Radiation Therapy Oncology Group; 2011.
- Ghia A, Tomé WA, Thomas S, et al. Distribution of brain metastases in relation to the hippocampus: implications for neurocognitive functional preservation. Int J Radiat Oncol Biol Phys 2007;68:971-7. [Crossref] [PubMed]
- Nevelsky A, Ieumwananonthachai N, Kaidar-Person O, et al. Hippocampal-sparing whole-brain radiotherapy using Elekta equipment. J Appl Clin Med Phys 2013;14:4205. [Crossref] [PubMed]
- Khan FM, Gibbons JP. Khan’s the physics of radiation therapy. 5th ed. Philadelphia, Pennsylvania: Lippincott Williams and Wilkins; 2014.
- Grégoire V, Mackie TR, De Neve W. Prescribing, recording, and reporting photon-beam intensity-modulated radiation therapy (IMRT): contents. J Int Comm Radiat Units Meas 2010;10:1-112.
- Moon SY, Yoon M, Chung M, et al. Comparison of the extent of hippocampal sparing according to the tilt of a patient’s head during WBRT using linear accelerator-based IMRT and VMAT. Phys Med 2016;32:657-63. [Crossref] [PubMed]
- Kumar SAS, George A. A study on the response of 2D ion chamber array detector for VMAT delivery. Int J Radiol Radiat Ther 2018;5:249-52. [Crossref]
- Gondi V, Tolakanahalli R, Mehta MP, et al. Hippocampal-sparing whole-brain radiotherapy: a “how-to” technique using helical tomotherapy and linear accelerator-based intensity-modulated radiotherapy. Int J Radiat Oncol Biol Phys 2010;78:1244-52. [Crossref] [PubMed]
- Krayenbuehl J, Di Martino M, Guckenberger M, et al. Improved plan quality with automated radiotherapy planning for whole brain with hippocampus sparing: a comparison to the RTOG 0933 trial. Radiat Oncol 2017;12:161. [Crossref] [PubMed]
- Huq MS, Das IJ, Steinberg T, et al. A dosimetric comparison of various multileaf collimators. Phys Med Biol 2002;47:N159-70.
- Wang S, Zheng D, Zhang C, et al. Automatic planning on hippocampal avoidance whole-brain radiotherapy. Med Dosim 2017;42:63-8. [Crossref] [PubMed]
- Kleiman NJ. Radiation cataract. Ann ICRP 2012;41:80-97. [Crossref] [PubMed]
- Nguyen SM, Sison J, Jones M, et al. Lens dose-response prediction modeling and cataract incidence in patients with retinoblastoma after lens-sparing or whole-eye radiation therapy. Int J Radiat Oncol Biol Phys 2019;103:1143-50. [Crossref] [PubMed]
- Dauer LT, Ainsbury EA, Dynlacht J, et al. Guidance on radiation dose limits for the lens of the eye: overview of the recommendations in NCRP Commentary No. 26. Int J Radiat Biol 2017;93:1015-23. [Crossref] [PubMed]
- Ezzell GA, Burmeister JW, Dogan N, et al. IMRT commissioning: multiple institution planning and dosimetry comparisons, a report from AAPM Task Group 119. Med Phys 2009;36:5359-73. [Crossref] [PubMed]
Cite this article as: Chen LJ, Li MH, Cheng HW, Kuo CY, Sun WL, Tsai JT. Hippocampus-sparing whole-brain radiotherapy: dosimetric comparison between non-coplanar and coplanar planning. Ther Radiol Oncol 2021;5:1.