Mitomycin C modulates tumor microenvironment and enhances radiosensitivity in rectal cancer
Introduction
Colorectal cancer (CRC) is a common malignancy which ranks third in incidence and second in cancer-related deaths globally (1). Neoadjuvant chemoradiation (CCRT) followed by surgery is the standard of care for locally advanced rectal cancer (2). However, only 10% to 25% of patients achieved pathological complete response (pCR) with neoadjuvant CCRT (3). Unsatisfactory pCR rates and potential toxicities remain the major challenge, moreover, the long-term clinical outcome is poor with 5-year overall survival and distant recurrence rates of 76% and 36% (4). These unmet medical needs require exploration of new strategies to increase the efficacy of current standard treatment with reduction of radiation toxicity.
Tumor hypoxia is one of the many molecular pathways and markers related to poor clinical outcome. It is responsible for development of aggressive phenotype and resistance to chemoradiation treatment (5,6). Hypoxia-inducible factor-1alpha (HIF-1α) manifests in solid tumors as they outgrow their local blood supply creating a hypoxic tumor microenvironment (TME) (7). Rasheed et al. showed that HIF-1α was expressed in greater than 50% of rectal cancer, which correlated with unfavorable prognosis (8). Besides the effect of hypoxia in upregulating tumor angiogenesis and promoting metastasis, a variety of immune cells in the TME affect the equilibrium of tumor immunity. Cytotoxic T lymphocytes (CTLs), natural killer (NK) cells, and dendritic cells (DCs) are crucial for pro-inflammatory cell killing while tumor infiltrating myeloid cell (TIMC) and regulatory T cells (Tregs) counteract with immunosuppression (9,10). Furthermore, tumor-associated macrophages (TAMs) can be M1 or M2 polarized and the tumor cell expression of ligands for PD-1, programmed cell death ligand 1 (PD-L1) within TME facilitates immune escape (11,12). To potentiate the therapeutic efficacy, use of a radiosensitizer with hypoxia activated feature may provide a novel strategy in targeting radioresistant hypoxic cells and alter the TME balance towards favorable anti-tumor immunity.
Mitomycin C (MMC) is known as a hypoxia-activated prodrug (HAP), which undergo bioreductive activation under hypoxia, translating into enhanced toxicity towards hypoxic tumor cells (5,6). Through altering redox status, MMC generates DNA-damaging species with antitumor profile (13). Chemoradiation with MMC is the standard of care for the treatment of anal cancer, however, MMC as an adjunct to radiotherapeutic management of rectal cancer is not well established (14). Theoretically, the combination of RT and MMC may present potential benefit of synergistic effect with possible change of intrinsic radiosensitivity in tumors. Currently, the effect of MMC on TME of irradiated rectal tumor is still unknown.
We aimed to investigate if MMC can radiosensitize CT26 adenocarcinoma cells in vitro and in vivo. In addition, we evaluated the combinatory effect of MMC and RT in modulating TME and enhancing the anti-tumor effect of rectal cancer.
Methods
Cell culture and hypoxic induction
For in vitro and in vivo experiments, BALB/c mice bearing CT26 colorectal adenocarcinoma cells were used. The cells were maintained in RPMI-1640 medium (GIBCO, Grand Island, NY, USA) supplemented with 10% heat-inactivated fetal calf serum (FCS) (Hyclone, Logan, UT, USA). In order to examine the role of MMC-induced radiosensitization under hypoxic condition, the cells were incubated in 1% O2 incubator for 2 hours prior to any type of treatment.
Cell viability assay
Tetrazolium dye 3-(4,5-dimethylthiazol-2-yl)-2,5-diphenylte-trazolium bromide (MTT) colorimetric method was used for cell viability assay. CT26 cells were cultured in 24-well plate at density 5×104 cells/mL. Various concentrations of MMC were administered over a period of 24 hours with 2 hours of hypoxic incubation. Tetrazolium salt converted to formazan and tetrazolium dye was added as an indicator. Spectrophotometer at 570 nm was used for measurement of formazan concentrations.
MMC treatment and radiation delivery in vitro
CT26 cells treated with 0, 0.1, 0.25, 0.5 µg/mL MMC and incubated for 2 hours in hypoxic condition for investigation in radiation sensitization. After washing, a linear accelerator (Clinac iX, Varian Associates, Inc., Palo Alto, CA; dose rate 4 Gy/min) was used to deliver 6 MeV of electron beam energy with various doses (0.5, 1, 2, and 4 Gy) in a single fraction. 24 hours after irradiation, the cells were collected and plated for colony formation assay.
Colony formation assay
102 viable tumor cells were coated in 6-well plate and grown in RPMI-1640 medium supplement with 10% of FCS at 37 °C in a 5% CO2 incubator. After 10 days of treatment, 0.4% crystal violet was used to stain the dishes and colonies (≥50 cells) were counted. Mean colonies/(cells inoculated x plating efficiency) represented the surviving fraction. Linear quadratic model was used to plot survival curves. Irradiation dose needed for RT alone divided by the dose needed for MMC plus RT at a surviving fraction of 37% was deemed the sensitizer enhancement ratio (SER).
MMC and RT effect on DNA damage repair
Cells were harvested at various times ranging from 0–24 hours after irradiation with 2 Gy and washed with PBS. Fixation and permeabilization was performed at 4 °C with ethanol (70%) for 1 h, incubated with Triton X-100 (1%), and stained with γ-H2AX antibodies (diluted 1:50; Cell Signaling Technology, Danvers, MA, USA). FACSCalibur flow cytometer (Becton Dickinson, Lincoln Park, NJ, USA) collected data from 104 cells ModFit software (Becton Dickinson, Lincoln Park, NJ, USA) was used for cell cycle analysis and calculation of the proportion of each phase.
Western blot
Phosphate-buffered saline (PBS) was used to wash treated cells and lysis buffer containing 20 mM Tris-HCl (pH7.5), 150 mM NaCl, 1 mM Na2EDTA, 1 mM EGTA, 1% triton, 2.5 mM sodium pyrophosphate, 1 mM β-glycerophosphate, 1 mM Na3VO4, 1 mg/mL leupeptin (Cell Signaling Technology, Danvers, MA, USA) for collection. Immunoblotting with primary antibodies HIF-1α (1:1,000; GeneTex, Irvine, CA, USA) was performed after using polyvinylidene fluoride membrane and blocking with 5% de-fatted milk. Horseradish peroxidase-labeled second antibodies (Jackson ImmunoResearch, West Grove, PA, USA) and enhanced chemiluminescence system (GeneTex, Irvine, CA, USA). Internal control was represented by the expression of beta-actin (Millipore, Burlington, MA, USA).
Interferon-β1 mRNA expression with reverse transcription and polymerase chain reaction
RNeasy mini kit (QIAGEN GmbH, Hilden, Germany) isolated total RNA from CT 26 cells. For further use in PCR, the total RNA was reverse-transcribed to cDNA. UniqueAssay ID qMmuCED0050444 for mouse Ifnb1 was the PrimePCR SYBR Green assay primers (BioRad) used in the study.
Animal model
Five-week-old male BALB/c mice were purchased from Animal Resource Center of the National Science Council of Taiwan (Taipei, Taiwan). Experimental Animal Committee of MacKay Memorial Hospital (approval number: MMH-A-S-106-01) approved all animal experimental protocols. Mice were fed with water and laboratory chow (Labdiet 5001; PMI Nutrition International LLC, St. Louis, MO) ad libitum. CT26 cells (4×106 cells/100 µL PBS) were subcutaneously implanted in the right hind leg of the mice. Tumors were grown for 7 days before treatment.
MMC administration and radiation delivery in vivo
Tumor cells were implanted into four groups of animals, each with 5 mice. The mice were randomly allocated to different treatment arms for two consecutive days: (I) no treatment (control); (II) fractionated radiation therapy (2 Gy × 2 fractions); (III) MMC 2 mg/kg body weight intraperitoneally (i.p.); (IV) fractionated radiotherapy (RT) (2 Gy × 2 fractions) in combination with MMC (2 mg/kg i.p., 2 h before irradiation). Zoletil 50 (25 mg/kg) plus Xylazine (10 mg/kg) prior to irradiation was used for anesthesia. The right hind leg was irradiated using the same linear accelerator with 2 Gy (6 MeV electron beam at source-to-surface distance of 100 cm, dose rate 4 Gy/min). MMC 2 mg/kg in vivo was chosen based on the conversion of clinically applied human dose (10 mg/m2) to mouse equivalent dose (15). 2 Gy per day was given for two consecutive days representing fractionated RT.
Evaluation of tumor volume, mouse weight, survival, and white blood cell count
Implanted tumor size and weight of mouse was measured every other day. Caliper was used to measure the largest (a) and smallest (b) diameters of tumor and volumes were estimated as 0.5ab2. For evaluation of systemic toxicity, the body weight recorded three times a week. Coulter counter (HEMAVET HV950; Drew Scientific, Inc., Dallas, TX, USA) was used to analyze the white blood cell (WBC) counts of the blood samples.
Measurement of immune cells with flow cytometry
Mice from each treatment groups were sacrificed 21 days after treatment for TME assessment. The mononuclear cells were purified before immunofluorescent staining for T cell markers, DCs, NK cells, myeloid-derived suppressor cells (MDSCs), tolerogenic (M2) TAMs, and T regulatory (Treg) cells. The whole spleen and tumor specimens were removed, cut in 2–4 mm pieces on ice and digested with solution containing Liberase TM (25 µg/mL) (Roche Diagnostics GmbH, Mannheim, Germany) and DNase I (10 µg/mL) (Sigma-Aldrich, St Louis, MO, USA) at 37 °C for 30 minutes. Single-cell suspensions were obtained with a 70-µm cell strainer. Incubation with ACK solution (Invitrogen, Waltham, MA, USA) was performed to lyse red blood cells. Before staining with cell surface markers, cells were resuspended in IgG from rat serum (1 µg/1×106 cells) (Sigma-Aldrich, St Louis, MO, USA) and incubated for 15 min at 4 °C to reduce nonspecific binding. Then, cells (1×106 cells/mL) were stained with antibodies conjugated with indicated fluorochromes for 30 min on ice, including anti-CD3 (PE), anti-NKG2D (PE), anti-CD11b (PE), anti-CD45 (PerCP), anti-CD206 (FITC), anti-CD8 (FITC), anti-CD83 (FITC), anti-MHCII (FITC), and anti-FoxP3 (APC; BioLegend). After staining, FACSCalibur flow cytometer (Becton Dickinson, Lincoln Park, NJ, USA) was used to evaluate infiltrated immune cells DCs (CD83+), M1 macrophages (MHC-II+), M2 macrophages (CD206+), NK cells (NKG2D+), and total T cells (CD3+). Detection of regulatory T cells (Tregs) staining with FoxP3 was performed according to the manufacture’s protocol using the True-NuclearTM Transcription Factor Staining Protocol for 5 mL Tubes (BioLegend, San Diego, CA, USA).
Immunohistochemical staining
Sections of formalin-fixed and paraffin-embedded mouse lymph nodes and tumors were treated with heat-induced antigen retrieval in sodium citrate buffer (10 mM sodium citrate, 0.05% Tween 20, pH 6.0) under 95−100 °C for 15 min, and followed with 3% H2O2 at room temperature for 10 min. BlockPROTM Blocking Buffer (Visual Protein, Taipei, Taiwan) at room temperature for 1 hour were used for blocking. Primary antibodies against PD-L1 (1:400; GeneTex, Irvine, USA) and HIF-1α (1:200; GeneTex, Irvine, USA) at room temperature for 1 hour, with secondary antibody of SignalStain® Boost IHC Detection Reagent (HRP, Rabbit) (Cell Signaling Technology, Danvers, MA, USA) at room temperature for 1 hour, and 5−10 minutes with DAB chromogen kit using EnVision™ + Dual Link System-HRP (Dako, Santa Clara, CA, USA) were used on the sections before counterstaining with hematoxylin (Merck, Kenilworth, NJ, USA). A pathologist and an oncologist with extensive experience on IHC assessment examined the expression of PD-L1 and HIF-1α.
Statistical analysis
All experiments were performed and repeated three times. Mean ± standard mean error from three independent experiments were used to express data. Statistical comparison of each experiment was performed with student’s t-test or one-way analysis of variance (ANOVA). Statistically significant values were indicated by P<0.05.
Results
Upregulation of HIF-1α expression in CT26 cells by hypoxia
To verify the establishment of hypoxic condition, CT26 cells were incubated in 1% oxygen incubator for 30 min, 1, 2, and 4 hours prior to treatment. Western blotting revealed increasing expression of HIF-1α after 2 hours of hypoxic incubation (Figure 1). The following in vitro experiments were performed with at least 2-h incubation prior to treatment to ensure upregulation of HIF-1α expression and hypoxic environment.
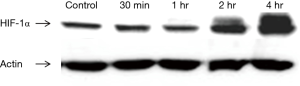
Effect of MMC and radiosensitization of CT26 cells in vitro
For cell viability, CT26 cells were pretreated with MMC and placed in a hypoxic incubator for 2 h. The highest non-toxic dose with treatment of MMC alone under hypoxia was 1.25 µg/mL (Figure 2A). To determine the MMC effects on radiation cell killing, radiation was added following MMC pretreatment and 2-h hypoxia induction. Cell killing was achieved with a lowered dose of MMC where pretreatment with 0.5 µg/mL MMC was sufficient to sensitize CT26 cells to radiation doses of 2 Gy and above (Figure 2B). The sensitizer enhancement ratio at 37% was 1.4.
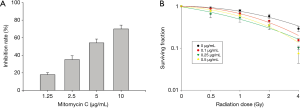
MMC pretreatment and radiation on DNA damage
Ionizing radiation or cytotoxic drugs induce DNA damage causing double stranded breaks (DSBs) (16). Following DNA damage, γ-H2AX rapidly forms at sites of DSBs to initiate DNA repair and response is maximal at 30 minutes post radiation exposure followed by decrease over time (17,18). We found that pretreatment with 5 µg/mL of MMC prior to 2Gy RT promoted the production and prolonged the reduction of γ-H2AX (Figure 3). Initial levels of γ-H2AX were increased with addition of MMC to RT and maintained over the course of 24 hours compared to RT treatment alone (P<0.05). This indicated that MMC promoted DNA DSBs and delayed DNA damage repair induced by RT.
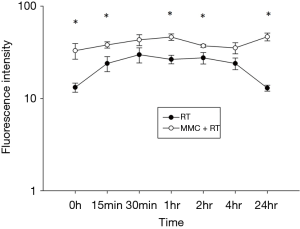
Interferon-β1 mRNA expression with reverse transcription and polymerase chain reaction
To assess the activation of anti-tumor immunity, interferon-β1 (Ifnb1) mRNA expression was evaluated via RT-PCR. MMC combined with 2 Gy of radiation showed a 1.8- or 3.4-fold increase in Ifnb1 mRNA expression compared to treatment with either RT or MMC alone, respectively (Figure 4).
MMC enhanced the effect of RT in vivo
CT26 tumor cells were implanted into the right hind legs and randomized into four treatment groups: (I) no treatment (control); (II) fractionated radiation therapy (2 Gy × 2 fractions); (III) MMC 2 mg/kg body weight intraperitoneally (i.p.); (IV) fractionated RT (2 Gy × 2 fractions) in combination with MMC (2 mg/kg i.p., 2 h before irradiation). The tumor volume decreased significantly from day 9 and onwards after treatment with MMC and RT (Figure 5A). Inhibition of tumor growth represents durable effect of combined treatment. Significant differences in body weight growth kinetics between the control and combined treatment groups were observed on day 21 (Figure 5B). There was no significant adverse effect on WBC counts between the different treatment groups (Figure 5C).
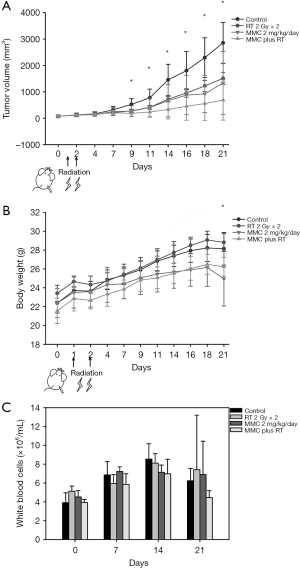
TME analysis
We next investigated TME using flow cytometry and analyzed the immune cells with corresponding surface markers: M1 (MHC-II+); M2 (CD206+); NK (NKG2D+); CTL (CD8+); DC (CD83+); tumor-infiltrating myeloid cell (TIMC) (CD11b+); total T cell (CD3+); Treg (FoxP3+) (Figures 6,7). RT increased intratumoral DCs by 1.2-fold compared to control, while MMC promoted M1 macrophages by 1.5-fold and total T cells by 2.8-fold in comparison to control (Figure 7). TIMC and M2 macrophage expression levels did not vary after treatment. Combined use of MMC with RT showed increased NK cells by 8.5-fold and CTLs by 4.8-fold compared to control (Figure 7). When combined treatment was weighed against RT treatment alone, an increase of NK cells by 9-fold and CTLs by 2-fold was observed (Figure 7). As for Tregs, decrease by half-fold in combination treatment group in comparison to control was noticed (Figure 7).
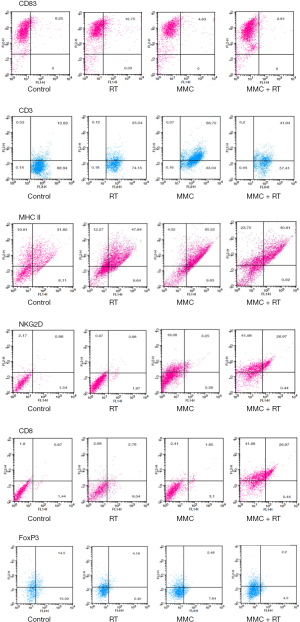
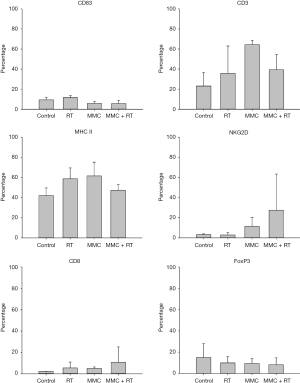
Immunohistochemistry and histopathological changes
In our in vivo experiment, immunohistochemistry (IHC) staining for HIF-1α was performed for tumor and lymph node sections. This was to verify the presence of hypoxic environment in the in vivo setting (Figure 8A,B).
To further investigate possible causes of tumor escape, immune checkpoint PD-L1 was stained. IHC results reveal increased expression of PD-L1 in tumor after treatment with combined MMC and RT in comparison to control (Figure 9).
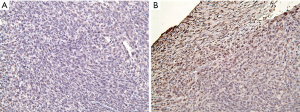
Discussion
This study revealed that MMC, a hypoxia activated prodrug (HAP), in combination with RT could enhance radioresponse and modulate TME toward inflamed phenotype. MMC demonstrated improve benefit when concurrently used with RT in the management of head and neck squamous cell cancer, but the effect on rectal cancer remains undetermined (19). The need for enhanced tumor radiosensitivity incited the use of MMC on mouse CT26 colorectal adenocarcinoma cells.
Drug repositioning is a concept to apply clinically accepted regents for new indications. The toxicity profile and management of adverse effect of MMC has been well established, including combined use with RT, which have possible risks of causing febrile neutropenia in 15% and leucopenia in 2% to 13% of patients (20). Our results revealed that MMC enhances the anti-tumor effect of RT against colorectal adenocarcinoma in vivo with inhibition of tumor growth and no significant toxicity or body weight loss (Figure 5). This implies safe therapeutic effect of MMC and a promising addition to RT in treatment of rectal cancer. Given that MMC has been widely used in CCRT of anal cancer with manageable toxicity such as moderate myelosuppression which can be prophylactically prevented or treated with colony stimulating factors, the combined administration of MMC and RT may be regarded as relatively safe (20,21). Taken together, this drug repositioning to CCRT of rectal cancer, other than anal cancer, might be reasonable and worthy of verification in the clinical setting.
We began by verifying presence of tumor hypoxia in both in vitro and in vivo setting as represented by upregulated HIF-1α expression (Figures 1 and 8). MMC enhanced the effect of RT as shown in radiation and drug survival assay (Figure 2). Although colon and rectal cancers demonstrate different entities with distinct treatment in clinical setting, the chemoradiosensitivity between colon and rectal cancer cell line does not differ significantly (22). This supports the translational value in our use of CT26 colorectal adenocarcinoma cells, suggesting MMC may be a novel candidate as a radiosensitizer for neoadjuvant CCRT in rectal cancer. In addition, the benefit of MMC in delaying DNA double-strand break repair up to 24 hours after irradiation compared to RT treatment alone, further establishes MMC as a potent radiosensitizer (Figure 3). The activity profile of MMC on targeting hypoxia and prolonging DNA damage repair may be the clue to the putative mechanism of radiosensitization in CT26 colorectal adenocarcinoma cells.
Recent finding revealed DNA damaging agents such as ionizing radiation can induce type I interferon and other cytokine expression (23). The cGAS-cGAMP-STING pathway is the gateway that connects DNA damage and innate immunity, however, STING signaling is found to be suppressed in colorectal carcinoma (24,25). We found that MMC plus RT further upregulated Ifnb1 mRNA expression compared to RT alone, suggesting possible enhanced activation of cGAS-cGAMP-STING pathway, which may have added effect in anti-tumor immunity (Figure 4). The outcome of anti-tumor response depends on the equilibrium variety of different immune cells, immune factors, and signaling molecules within the TME (10). Therefore, other than being radiosensitizer acting on local hypoxic tumor sites, the immune modulating activity of MMC and RT in the TME of rectal cancer prompted further interest for investigation. Our findings showed that RT promoted intratumoral DCs, which was consistent with previous studies on enhanced anti-tumor immunity of radiation inducing DCs (Figures 6,7) (26,27). The addition of MMC did not augment DC immune response, rather, MMC had more effect on increasing M1 macrophages and total T cells (Figures 6,7). This implicated that although the effector cell ontogeny modulated by MMC might not be DCs, both ionizing radiation and MMC enhanced antigen presenting cells of different phenotypes. In comparison to either RT or MMC treatment as a single modality, the effect in the combination of MMC and fractionated RT is more appealing. Combined treatment markedly recruited CTL and NK cells into tumor and reduced infiltration of Treg, indicating a favorable immunomodulating effect (Figures 6,7). Part of the effect might be due to ionizing radiation inducing stronger proliferation of effector T cells rather than Treg as discovered by Persa et al. (28).
The density in infiltration of lymphocytes and presence of both NK and CD8 cells in the CRC microenvironment represents a favorable prognostic impact that is associated with better overall survival in CRC (29,30). This is due to the enhanced expression of NKG2D ligands, which promotes antitumor response as a result of DNA damage (31,32). In addition, Treg depletion is known to increase NK cell functions and proliferation (33). The reduction of Treg by MMC and RT in our study may render the increase in NKG2D+ NK cells responsible for synergizing activation of anti-tumoral immunity.
Intriguingly, the generation of active alkylating metabolites of MMC in hypoxic niche within tumor to modulate TME is a unique finding (13). MMC in our study reversed the impairment of T cell infiltration in the hostile TME created by hypoxic stress (34). MMC has the advantages of targeting local intratumoral hypoxia and also escalating intercellular immune response signaling from a local to systemic level. Unlike other immunomodulators acting systemically, HAP such as MMC modifies biological response locally and generates in situ immunomodulation in the target tumor. This may provide a novel strategy to develop potent immune booster without interference of pharmacokinetics and pharmacodynamics.
Modulation of TME toward favorable immune-cell lineage commitment with combination treatment of MMC and RT indicate beneficial anti-tumor response. We found that combinatory treatment enhanced PD-L1 expression in tumor (Figure 9). Since PD-L1 on tumor cells or immune host cells are involved in immune evasion and preventing T cell-mediated killing, this implicates use of immune checkpoint blockade such as anti-PD-L1 for beneficial outcome (35,36). Noguchi et al. demonstrated PD-L1 on tumor cells can be upregulated when stimulated by interferons, yet, the major contributor of PD-L1 expression is from TAMs on immune host cells (37). Furthermore, TAMs with upregulated PD-1 and PD-L1 expresses M2 polarization which facilitates immune escape (11,12). Our results show that MMC has the added benefit of polarizing TAMs towards M1 anti-tumoral immunity (Figures 6,7). One can speculate that with increase in infiltration of immune cells and PD-L1 expression on tumor after treatment via MMC plus RT, the combination of immune checkpoint blockade renders an attractive modality to enhance tumor recognition and cancer cell elimination in the inflamed niche.
While it is already known that RT can induce immune cell infiltration, our study demonstrated the addition of MMC augmented anti-tumor immunity to an even greater extent, certifying MMC as an effective radiosensitizer. Low and high dose irradiation act on different pathways in DCs, yet the optimal radiation dose is unexplored in the current study (28). Limitation remains in determining target effector cells and further investigation is required into PD-1/PD-L1 blockade. The causal relationship between cGAS-STING-Interferon type I signaling pathway and the stimulated inflammatory cytokine expression needs further clarification. Upregulated interferon plays important role in regulating immune cell infiltration, as evident with the altercation in TME after treatment. Whether this immunomodulatory effect correlates to increase immune cell infiltration and decreased tumor evasion remains to be elucidated. Further investigation is necessary in identifying biomarkers for hypoxia since it is present in greater than 50% but not all CRC (8). Hypoxic biomarkers are important to determining patient subgroups expected to benefit most from treatment with HAP and RT. Frey et al. discovered that antitumor immune responses are timely restricted suggesting variable days of high and low immune cell infiltration (27). Thus, the chronological order of drug and RT administration for synergistic effect may be further studied during the design of multimodal radioimmunotherapies. Our preclinical data on combined treatment of MMC and RT and its safe use with attraction of immune cells into CRC tumors and enhancement of PD-L1 expression in the TME paves the path for future clinical protocols. The translational aspect is valuable for the optimization of MMC and RT with further design of multimodality treatment to include immunotherapy for the improvement in clinical treatment outcomes of CRC.
Conclusions
Our results implicate the combination of MMC and conventional fractionated RT may have synergistic therapeutic effect accompanied by modulation of TME toward favorable immune-cell lineage commitment. The correlation between impaired DNA repair, upregulated Ifnb1 mRNA expression, and TME alteration requires additional clarification. Further optimization for combination of MMC and conventional fractionated RT is warranted before translating to clinical research.
Acknowledgments
Funding: This study was supported by MacKay Memorial Hospital (grant number MMH-E-107-13 and MMH-E-108-13) and the Ministry of Science and Technology, Taiwan (grant number MOST 106-2314-B-195-002-MY3).
Footnote
Conflicts of Interest: YJC serves as an Editors-in-Chief of Therapeutic Radiology and Oncology. The other authors have no conflicts of interest to declare.
Ethical Statement: The authors are accountable for all aspects of the work in ensuring that questions related to the accuracy or integrity of any part of the work are appropriately investigated and resolved. All experimental protocols involving animals were reviewed and approved by the Experimental Animal Committee of MacKay Memorial Hospital (approval number: MMH-A-S-106-01).
Open Access Statement: This is an Open Access article distributed in accordance with the Creative Commons Attribution-NonCommercial-NoDerivs 4.0 International License (CC BY-NC-ND 4.0), which permits the non-commercial replication and distribution of the article with the strict proviso that no changes or edits are made and the original work is properly cited (including links to both the formal publication through the relevant DOI and the license). See: https://creativecommons.org/licenses/by-nc-nd/4.0/.
References
- Bray F, Ferlay J, Soerjomataram I, et al. Global cancer statistics 2018: GLOBOCAN estimates of incidence and mortality worldwide for 36 cancers in 185 countries. CA Cancer J Clin 2018;68:394-424. [Crossref] [PubMed]
- Bosset JF, Collette L, Calais G, et al. Chemotherapy with preoperative radiotherapy in rectal cancer. N Engl J Med 2006;355:1114-23. [Crossref] [PubMed]
- Dossa F, Acuna SA, Rickles AS, et al. Association Between Adjuvant Chemotherapy and Overall Survival in Patients With Rectal Cancer and Pathological Complete Response After Neoadjuvant Chemotherapy and Resection. JAMA Oncol 2018;4:930-7. [Crossref] [PubMed]
- Sauer R, Becker H, Hohenberger W, et al. Preoperative versus Postoperative Chemoradiotherapy for Rectal Cancer. New England Journal of Medicine 2004;351:1731-40. [Crossref] [PubMed]
- Phillips RM. Targeting the hypoxic fraction of tumours using hypoxia-activated prodrugs. Cancer Chemother Pharmacol 2016;77:441-57. [Crossref] [PubMed]
- Mistry IN, Thomas M, Calder EDD, et al. Clinical Advances of Hypoxia-Activated Prodrugs in Combination With Radiation Therapy. Int J Radiat Oncol Biol Phys 2017;98:1183-96. [Crossref] [PubMed]
- Ioannou M, Paraskeva E, Baxevanidou K, et al. HIF-1alpha in colorectal carcinoma: review of the literature. J buon 2015;20:680-9. [PubMed]
- Rasheed S, Harris AL, Tekkis PP, et al. Hypoxia-inducible factor-1alpha and -2alpha are expressed in most rectal cancers but only hypoxia-inducible factor-1alpha is associated with prognosis. Br J Cancer 2009;100:1666-73. [Crossref] [PubMed]
- Gajewski TF, Schreiber H, Fu YX. Innate and adaptive immune cells in the tumor microenvironment. Nat Immunol 2013;14:1014-22. [Crossref] [PubMed]
- Pitt JM, Marabelle A, Eggermont A, et al. Targeting the tumor microenvironment: removing obstruction to anticancer immune responses and immunotherapy. Ann Oncol 2016;27:1482-92. [Crossref] [PubMed]
- Sica A, Schioppa T, Mantovani A, et al. Tumour-associated macrophages are a distinct M2 polarised population promoting tumour progression: potential targets of anti-cancer therapy. Eur J Cancer 2006;42:717-27. [Crossref] [PubMed]
- Gordon SR, Maute RL, Dulken BW, et al. PD-1 expression by tumour-associated macrophages inhibits phagocytosis and tumour immunity. Nature 2017;545:495-9. [Crossref] [PubMed]
- Kennedy KA, Rockwell S, Sartorelli AC. Preferential activation of mitomycin C to cytotoxic metabolites by hypoxic tumor cells. Cancer Res 1980;40:2356-60. [PubMed]
- Shridhar R, Shibata D, Chan E, et al. Anal cancer: current standards in care and recent changes in practice. CA Cancer J Clin 2015;65:139-62. [Crossref] [PubMed]
- Nair AB, Jacob S. A simple practice guide for dose conversion between animals and human. J Basic Clin Pharm 2016;7:27-31. [Crossref] [PubMed]
- Paull TT, Rogakou EP, Yamazaki V, et al. A critical role for histone H2AX in recruitment of repair factors to nuclear foci after DNA damage. Curr Biol 2000;10:886-95. [Crossref] [PubMed]
- Sedelnikova OA, Horikawa I, Zimonjic DB, et al. Senescing human cells and ageing mice accumulate DNA lesions with unrepairable double-strand breaks. Nat Cell Biol 2004;6:168-70. [Crossref] [PubMed]
- Rogakou EP, Boon C, Redon C, et al. Megabase chromatin domains involved in DNA double-strand breaks in vivo. J Cell Biol 1999;146:905-16. [Crossref] [PubMed]
- Haffty BG, Wilson LD, Son YH, et al. Concurrent chemo-radiotherapy with mitomycin C compared with porfiromycin in squamous cell cancer of the head and neck: final results of a randomized clinical trial. Int J Radiat Oncol Biol Phys 2005;61:119-28. [Crossref] [PubMed]
- Ajani JA, Winter KA, Gunderson LL, et al. Fluorouracil, mitomycin, and radiotherapy vs fluorouracil, cisplatin, and radiotherapy for carcinoma of the anal canal: a randomized controlled trial. Jama 2008;299:1914-21. [Crossref] [PubMed]
- Flam M, John M, Pajak TF, et al. Role of mitomycin in combination with fluorouracil and radiotherapy, and of salvage chemoradiation in the definitive nonsurgical treatment of epidermoid carcinoma of the anal canal: results of a phase III randomized intergroup study. J Clin Oncol 1996;14:2527-39. [Crossref] [PubMed]
- Spitzner M, Emons G, Kramer F, et al. A gene expression signature for chemoradiosensitivity of colorectal cancer cells. Int J Radiat Oncol Biol Phys 2010;78:1184-92. [Crossref] [PubMed]
- Deng L, Liang H, Xu M, et al. STING-Dependent Cytosolic DNA Sensing Promotes Radiation-Induced Type I Interferon-Dependent Antitumor Immunity in Immunogenic Tumors. Immunity 2014;41:843-52. [Crossref] [PubMed]
- Li T, Chen ZJ. The cGAS-cGAMP-STING pathway connects DNA damage to inflammation, senescence, and cancer. J Exp Med 2018;215:1287-99. [Crossref] [PubMed]
- Xia T, Konno H, Ahn J, et al. Deregulation of STING Signaling in Colorectal Carcinoma Constrains DNA Damage Responses and Correlates With Tumorigenesis. Cell Rep 2016;14:282-97. [Crossref] [PubMed]
- Teitz-Tennenbaum S, Li Q, Okuyama R, et al. Mechanisms involved in radiation enhancement of intratumoral dendritic cell therapy. J Immunother 2008;31:345-58. [Crossref] [PubMed]
- Frey B, Ruckert M, Weber J, et al. Hypofractionated Irradiation Has Immune Stimulatory Potential and Induces a Timely Restricted Infiltration of Immune Cells in Colon Cancer Tumors. Front Immunol 2017;8:231. [Crossref] [PubMed]
- Persa E, Szatmári T, Sáfrány G, et al. In Vivo Irradiation of Mice Induces Activation of Dendritic Cells. Int J Mol Sci 2018; [Crossref] [PubMed]
- Pérez-Ruiz E, Berraondo P. Immunological Landscape and Clinical Management of Rectal Cancer. Front Immunol 2016;7:61. [Crossref] [PubMed]
- Sconocchia G, Eppenberger S, Spagnoli GC, et al. NK cells and T cells cooperate during the clinical course of colorectal cancer. Oncoimmunology 2014;3:e952197 [Crossref] [PubMed]
- Bauer S, Groh V, Wu J, et al. Activation of NK Cells and T Cells by NKG2D, a Receptor for Stress-Inducible MICA. Science 1999;285:727-9. [Crossref] [PubMed]
- Gasser S, Orsulic S, Brown EJ, et al. The DNA damage pathway regulates innate immune system ligands of the NKG2D receptor. Nature 2005;436:1186-90. [Crossref] [PubMed]
- Pedroza-Pacheco I, Madrigal A, Saudemont A. Interaction between natural killer cells and regulatory T cells: perspectives for immunotherapy. Cell Mol Immunol 2013;10:222-9. [Crossref] [PubMed]
- Chouaib S, Noman MZ, Kosmatopoulos K, et al. Hypoxic stress: obstacles and opportunities for innovative immunotherapy of cancer. Oncogene 2017;36:439-45. [Crossref] [PubMed]
- Tang F, Zheng P. Tumor cells versus host immune cells: whose PD-L1 contributes to PD-1/PD-L1 blockade mediated cancer immunotherapy? Cell Biosci 2018;8:34. [Crossref] [PubMed]
- Zou W, Wolchok JD, Chen L. PD-L1 (B7-H1) and PD-1 pathway blockade for cancer therapy: Mechanisms, response biomarkers, and combinations. Sci Transl Med 2016;8:328rv4 [Crossref] [PubMed]
- Noguchi T, Ward JP, Gubin MM, et al. Temporally Distinct PD-L1 Expression by Tumor and Host Cells Contributes to Immune Escape. Cancer Immunol Res 2017;5:106-17. [Crossref] [PubMed]
Cite this article as: Chen FYS, Chi CW, Shieh HR, Lin CP, Ko CC, Chung YC, Lai JCY, Tai HC, Chen YJ. Mitomycin C modulates tumor microenvironment and enhances radiosensitivity in rectal cancer. Ther Radiol Oncol 2019;3:29.