A planning study for evaluation of feasibility and benefit of generalized equivalent uniform dose-assisted optimization in volumetric-modulated arc therapy for recurrent head and neck cancer
Introduction
Radiation therapy plays an important role in the multi-modality treatments strategy for head and neck cancer (HNC), either in the post-operative or definitive setting. Despite the advances in management of HNC, failure to the initial treatments for locally advanced disease resulting in local or loco-regional recurrence still happens to up to 40% of patients (1-3). Traditionally, salvage surgery would be the most effective curative intent treatment option for patients with resectable tumors and sufficient good performance status, however, which account for only a select minority. Re-irradiation to the previously treated area is the possible alternative but poses great challenges to balancing tumor control and late normal tissue toxicity such as extensive fibrosis, osteoradionecrosis, carotid artery blowout and myelopathy. Because of the advent of enormous progress of radiation therapy technology, sophisticated irradiation technique such as intensity-modulated radiotherapy (IMRT) and volumetric-modulated arc therapy (VMAT), which are capable of providing highly conformal dose distribution with better critical structures sparing makes re-irradiation more acceptable nowadays.
Several reports (2-5) showed that IMRT is more effective and safer for re-irradiation of recurrent HNC compared to conventional technique since that dose escalation could be achievable without exceeding the tolerance of surrounding pre-irradiated late-reacting tissues. Lee et al. (5) retrospectively evaluated a re-irradiation series and revealed a significant increased loco-regional progression free survival in multivariate analysis for patients re-irradiated with IMRT approach.
As a novel radiation technique, VMAT could provide equivalent target volume coverage, dose conformity and homogeneity as well as non-inferior organs at risk (OARs) sparing with additional advantage of reduced monitor units (MUs) and shortened treatment delivery time in many cancer sites (6). More and more studies (7) investigated the applicability of VMAT for HNC using different treatment planning systems (TPSs), which revealed that two arc VMAT plans achieved better homogeneity, similar conformity with slightly improved sparing of the spinal cord (SC), brain stem, and contra-lateral parotid gland compared to IMRT. Although there is a paucity of data for applying VMAT in re-irradiation setting, it would be considered as a reasonable and promising technique based on an extrapolation of the above evidence.
Conventionally, physical constraints are used, namely, dose-volume (DV) parameters in inverse treatment planning. However, the DV-based objective functions cannot truly reflect the relationship between the biological reactions of tumors, OARs and the radiation dose. The introduction of biological objectives such as generalized equivalent uniform dose (gEUD), tumor control probability (TCP) and normal tissue complication probability (NTCP) in planning optimization process gained increasing attention over the last decade. Niemierko (8) proposed the concept of equivalent uniform dose (EUD), a single metric for reporting non-uniform tumor dose distribution, and further extended it to gEUD (9), which is a single organ specific parameter to account for the biological response according to the delivered dose distribution in that organ. Three commercially available and most commonly used TPSs, namely, Monaco®, Pinnacle®, and Eclipse, offer biological optimization features in their inverse treatment planning module, and gEUD objective is adopted due to which incorporates information about the organ functional architecture serial or parallel, lies in a DV domain more familiar to clinicians, and possesses desired mathematical properties. A few researches (10-16) regarding IMRT incorporating gEUD constraints have demonstrated that biological optimization, either gEUD-based or hybrid, namely, combined DV-based with gEUD cost functions often leads to improved sparing of OARs with comparable target coverage compared to physical optimization alone. However, limited planning studies (17,18) assessing applying gEUD objective in VMAT optimization were published to date.
SC is one of the most critical OARs during radiotherapy. For receiving radiation doses of 50 and 60 Gy, the risk of myelopathy is 0.2%, and 6%, respectively (19). Although that SC tolerance recovery happens to some extent after an elapsed period of time is found in some literature reviews (20,21), the need for sparing of additional dose to SC when re-irradiation as low as technically possible is still paramount to reduce risk of myelopathy.
Hence, the aim of our study was to investigate the feasibility of incorporating the biologically based gEUD objective function in the treatment planning optimization process for cases with recurrent HNC treated with re-irradiation using VMAT to see if any further benefit could be found with respect to its potential to improve dose sparing of the OARs, focusing on the SC, without compromising dose coverage of planning target volume (PTV). We selected different locations and varied sizes of PTV of recurrent HNC for the purpose of hoping to figure out the possible factors that could affect the dosimetric outcomes when using gEUD-assisted optimization.
Methods
Five recurrent HNC cases with prior radiotherapy history were selected from our department database. No matter what kind of re-irradiation technique used in actual treatment, new VMAT plans were generated for each case in this planning study. In order to simulate the clinical situation of diversely nature, five different tumor sites and volumes were chosen. The characteristics of these cases such as volume of PTV and distance between PTV and SC were listed in Table 1.
Table 1
Characteristics | Case 1 | Case 2 | Case 3 | Case 4 | Case 5 |
---|---|---|---|---|---|
PTV volume (cc) | 63.4 | 81.8 | 199.7 | 86.4 | 86.1 |
Distance between PTV and SC (cm) | 3.5 | 3.0 | 2.5 | 1.6 | 1.2 |
Arc angle (°) | 240-70 | 320-120 | 210-50 | 181-340 | 200-30 |
PTV, planning target volume; SC, spinal cord.
The same attending physician specializing in treating HNC delineated the target volume and OARs for all cases, so that the impact of interoperator variations on the contouring was diminished. The OARs for evaluation in this study included SC and contralateral parotid gland due to that all the recurrent tumor chosen located unilaterally either left-sided or right-sided. Other OARs such as brain stem, eyes, lens, optic nerves, chiasm, inner ears, cochleas and larynx were also contoured. The PTV in each case comprised gross contrast enhancing recurrent tumor region with an evenly expanded margin of 5 mm in 6 axes.
All VMAT plans were created using the same 6 MV photon beams commissioned for an Elekta Versa HDTM Linac (Elekta, Stockholm, Sweden) and were performed with Philips Pinnacle3® Planning System v. 9.8 (Philips Medical Systems, Fitchburg, WI, USA) using the SmartArc module with collapsed-cone convolution algorithm with a calculation resolution of 3 mm. Every VMAT plan consists of three repetitive partial arcs with an appropriate gantry rotation range determined corresponding to the PTV location, extent and relation with SC. The directions of two duplicate partial arcs were counter clockwise and the other one was clockwise. The start and stop angles used in each case were depicted in Table 1. For optimization between calculation time and accuracy of treatment planning, the final dose was calculated at a gantry angle spacing of 4°. The same experienced medical physicist carried out all these plans to avoid bias resulted from interoperator difference.
Pinnacle3® is not a designated biologically based optimization system, but rather applies biological cost functions to enhance the traditional, DV-based optimization method. In addition to a number of DV cost functions such as Min dose, Max dose, Uniform dose, Min DVH, Max DVH, and Uniformity, Pinnacle3® has three biological cost functions named Min EUD, Target EUD, and Max EUD at its disposal. The Target EUD and Min EUD can be selected for the PTV, while Max EUD is used for OAR.
The gEUD is a phenomenological formula defined as below:
where vi is the fractional organ volume receiving a dose Di and ɑ is a parameter that denotes the volume effect. For ɑ → −ꝏ (negative ɑ values, down to −50 is available in practice), the gEUD approaches the minimum dose, and can be used for target volumes. When ɑ =1, the gEUD equals the mean dose, and could be used in place of the mean objective for parallel organs. For ɑ >1 (high ɑ values, up to 50 in practice), the gEUD weights more on the high-dose area, normally used to drive high (maximum) dose of serial organs.
Based on the study published by Lee et al. (11) which concluded that starting with a DV-generated plan and then improving it by adding gEUD-based optimization, can reduce the number of iterations and also improve the optimum dose distribution, we conducted our planning study in two phases described as below:
The first phase
Run the DV-based optimization to generate a DV plan with respect to the DV criteria. The dose/fractionation prescribed for the PTV in our study was 60 Gy in 30 fractions. With regard to the OARs, dose constraints were set as follows: the maximum dose (Dmax) to the SC and brain stem were both set as 6 Gy with the consideration of remained tolerance of the pre-irradiated tissue; the mean dose (Dmean) to the lens was set as 6 Gy. No constraint was set for parotid glands in this study. The dose to remaining normal tissues was minimized within a reasonable range without affecting the target coverage [Ring <54 Gy and unspecified imaged volume (UIV) <30 Gy]. The two main objectives set to achieve were V100% ≥95% for PTV, namely, 100% of prescription dose would encompass at least 95% volume of the PTV and Dmax of SC <6 Gy. DV optimization was run repeatedly until the two objectives fulfilled strictly.
The second phase
Based on the original DV plan created in the first phase, we added the Max EUD constraint for SC to evaluate the impact of incorporating single biologically based gEUD objective function on the plan quality regarding the target dose coverage and OAR sparing. In order to investigate an approach about choosing the proper Max EUD constraint for the SC and to test the limit value, we used the gEUD value of the SC calculated from DV plan as a reference, then adopted 90% of this value (named SC_gEUD90%) to set the Max EUD constraint of the SC for further optimization to generate a new DV-gEUD plan. We repeated the same method by changing Max EUD constraint of the SC to SC_gEUD80%, SC_gEUD70% and so on to create respective new DV-gEUD plans till the criterion V100% ≥95% for PTV unmet. Since that the SC is a typical serial organ, a fixed parameter ɑ value of 20 was adopted per report of AAPM Task Group 166 (22). No interactions were allowed during the second phase optimization process.
We evaluated the extent of plan quality improvement after gEUD-assisted optimization by analyzing and comparing the dosimetric parameters between the original DV plans and respective DV-gEUD plans adopting specific SC Max EUD constraint.
Evaluation parameters
- PTV dose coverage: PTV V100%, namely, the percentage of the target volume receiving the 100% of prescription dose as displayed on the cumulative dose-volume histogram (DVH) was reported for each plan;
- Conformity index (CI): a ratio used for evaluation of the fitness of the prescription isodose volume to the PTV in treatment plans. We adopted the formula defined by RTOG (23) as VRI/TV, where VRI means reference isodose volume, and TV means target volume. The CI is usually situated between 1 and 2; when the CI approached 1, the ideal coverage achieved;
- Homogeneity index (HI): a ratio used for assessing dose homogeneity within the PTV. We adopted the RTOG definition of HI determined as Dmax located in the treatment volume divided by the prescription dose (Dp), with a value closer to 1 indicating better homogeneity.
- OAR dose distribution: we analyzed the Dmax and gEUD of SC, and Dmean of the contralateral parotid gland for all plans.
Statistical analyses
We chose paired t-test to compare the dosimetric differences in original DV plans versus respective DV-gEUD plans when acceptable DV-gEUD plan adopting the same level of specific SC Max EUD constraint were created for all the five cases. A two-tailed P value <0.05 was considered to be significant statistically. The statistical software PASW® Statistics 18 was used for data analyzing.
Results
A total of 24 plans for evaluation including five original DV plans in the first phase and 19 DV-gEUD plans composed of 3–4 plans for each case in the second phase were created. The mean volume of PTV was 103.38 cm3 (63.40–199.70 cm3). All the DV plans fulfilled the acceptable criteria as PTV V100% ≥95% and SC Dmax <6 Gy. The acceptable target coverage criterion was met in all DV-gEUD plans using SC_gEUD90% and SC_gEUD80% as Max EUD constraint of SC except in case 5 with the closest distance between PTV and SC of only 12 mm.
The results of dosimetric parameters regarding PTV dose distribution and SC sparing, such as PTV V100%, the standard deviation (SD) inside the target and Dmax of SC for all DV and DV-gEUD plans were summarized in Table 2. The isodose curve distribution and DVHs of the DV plan and DV-gEUD plan for case 1 is displayed in Figure 1. Better SC sparing as reducing Dmax of SC to varied extent could be achieved by DV-gEUD optimization adopting stepwise stricter Max EUD constraints within individual case comparison with an exception of using SC_gEUD70% in case 5. A trend of that the nearer of the distance between PTV and SC, the less benefit of SC sparing provided by gEUD-assisted optimization could be observed from Figure 2.
Table 2
Plan name | Case 1 | Case 2 | Case 3 | Case 4 | Case 5 | ||||||||||||||
---|---|---|---|---|---|---|---|---|---|---|---|---|---|---|---|---|---|---|---|
Tg SD | V100% (%) | SC Dmax (cGy) | Tg SD | V100% (%) | SC Dmax (cGy) | Tg SD | V100% (%) | SC Dmax (cGy) | Tg SD | V100% (%) | SC Dmax (cGy) | Tg SD | V100% (%) | SC Dmax (cGy) | |||||
DV | 0.99 | 99.0 | 372.9 | 1.04 | 98.4 | 308.9 | 1.23 | 98.0 | 379.2 | 2.68 | 96.7 | 522.1 | 2.43 | 96.5 | 478.1 | ||||
SC_gEUD95% | N/A | N/A | N/A | N/A | N/A | N/A | N/A | N/A | N/A | N/A | N/A | N/A | 3.18 | 94.7 | 458.1 | ||||
SC_gEUD90% | 1.21 | 98.0 | 232.5 | 1.81 | 97.4 | 229.5 | 1.58 | 97.0 | 362.1 | 2.69 | 97.5 | 427.5 | 3.20 | 94.6 | 456.8 | ||||
SC_gEUD80% | 1.46 | 97.5 | 218.0 | 1.99 | 96.3 | 227.4 | 1.96 | 95.0 | 319.3 | 2.70 | 97.0 | 424.1 | 3.43 | 92.0 | 453.7 | ||||
SC_gEUD75% | N/A | N/A | N/A | 2.84 | 89.7 | 240.4 | 1.98 | 91.0 | 308.8 | 2.81 | 96.0 | 410.3 | N/A | N/A | N/A | ||||
SC_gEUD70% | 1.62 | 96.5 | 212.8 | N/A | N/A | N/A | 2.08 | 91.0 | 305.1 | 2.82 | 96.0 | 412.4 | 3.74 | 89.8 | 506.3 | ||||
SC_gEUD60% | 1.67 | 97.0 | 198.2 | N/A | N/A | N/A | N/A | N/A | N/A | N/A | N/A | N/A | N/A | N/A | N/A |
DV, dose-volume; gEUD, generalized equivalent uniform dose; Tg SD, the standard deviation (in Gy) inside the target; V100%, the percentage of the target volume receiving the prescription dose; SC Dmax, the maximum dose of spinal cord; SC_gEUD90%, 90% of the gEUD value of the spinal cord calculated from DV plan, and so on; N/A, not available.
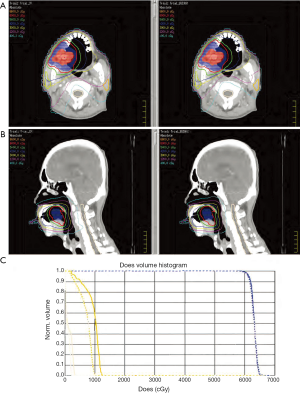
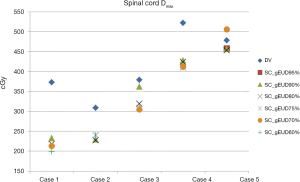
When adopting SC_gEUD90% and SC_gEUD80% as Max EUD constraint of SC, DV-gEUD optimization reduced the average Dmax of SC calculated from all cases by 17.1% (mean difference 70.6±52.0 cGy, P=0.039) and 20.3% (mean difference 83.7±48.4 cGy, P=0.018), respectively compared to DV optimization with statistical significance. After excluding cases with largest volume of PTV and shortest distance between PTV and SC, the reductions were 26.1% and 27.8%, respectively.
Table 3 demonstrated the relationship between the Max EUD set and the corresponding gEUD calculated of the SC and indicated that there would be an ultimate limit of gEUD. Further restriction of Max EUD objective might sacrifice the PTV coverage rather than reduce Dmax of the SC, especially in case with short distance between target and OAR.
Table 3
Plan name | Case 1 | Case 2 | Case 3 | Case 4 | Case 5 | |||||||||
---|---|---|---|---|---|---|---|---|---|---|---|---|---|---|
EUD (cGy) | gEUD (cGy) | EUD (cGy) | gEUD (cGy) | EUD (cGy) | gEUD (cGy) | EUD (cGy) | gEUD (cGy) | EUD (cGy) | gEUD (cGy) | |||||
DV | N/A | 291 | N/A | 242 | N/A | 303 | N/A | 394 | N/A | 360 | ||||
SC_gEUD95% | N/A | N/A | N/A | N/A | N/A | N/A | N/A | N/A | 342 | 349 | ||||
SC_gEUD90% | 262 | 188 | 218 | 181 | 273 | 272 | 355 | 324 | 324 | 348 | ||||
SC_gEUD80% | 233 | 174 | 194 | 175 | 243 | 241 | 315 | 320 | 288 | 347 | ||||
SC_gEUD75% | N/A | N/A | 182 | 186 | 228 | 235 | 296 | 312 | N/A | N/A | ||||
SC_gEUD70% | 204 | 169 | N/A | N/A | 213 | 231 | 276 | 314 | 252 | 380 | ||||
SC_gEUD60% | 175 | 161 | N/A | N/A | N/A | N/A | N/A | N/A | N/A | N/A |
DV, dose-volume; EUD, equivalent uniform dose; gEUD, generalized equivalent uniform dose; SC_gEUD90%, 90% of the gEUD value of the spinal cord calculated from DV plan, and so on; N/A, not available.
Regarding the conformity of PTV evaluated by CI, the results were similar between DV and corresponding DV-gEUD plans in three of the five cases (cases 1, 2 and 4) as shown in Table 4 and Figure 3. There was a trend of improved CI towards 1 in the other two cases with large volume of PTV and short distance between PTV and SC, however, compromised PTV coverage was an unsatisfactory trade-off. Since that the high-dose spillage is likely the culprit in most of the serial-organ toxicities, confining the high dose region to fit the defined target volume with an acceptable dose coverage would be advantageous in such a challenging situation with heavily pre-irradiated surrounding tissues.
Table 4
Plan name | Case 1 | Case 2 | Case 3 | Case 4 | Case 5 | ||||||||||||||
---|---|---|---|---|---|---|---|---|---|---|---|---|---|---|---|---|---|---|---|
HI | CI | Contralateral parotid Dmean (cGy) | HI | CI | Contralateral parotid Dmean (cGy) | HI | CI | Contralateral parotid Dmean (cGy) | HI | CI | Contralateral parotid Dmean (cGy) | HI | CI | Contralateral parotid Dmean (cGy) | |||||
DV | 1.1033 | 1.3783 | 945 | 1.0870 | 1.2570 | 463 | 1.1304 | 1.2534 | 700 | 1.1145 | 1.1139 | 142 | 1.1144 | 1.2332 | 205 | ||||
SC_gEUD95% | N/A | N/A | N/A | N/A | N/A | N/A | N/A | N/A | N/A | N/A | N/A | N/A | 1.1399 | 1.1243 | 188 | ||||
SC_gEUD90% | 1.1066 | 1.3739 | 707 | 1.1068 | 1.3217 | 267 | 1.1341 | 1.1041 | 579 | 1.1190 | 1.1656 | 116 | 1.1384 | 1.1161 | 189 | ||||
SC_gEUD80% | 1.1111 | 1.3692 | 585 | 1.1013 | 1.2482 | 233 | 1.1542 | 1.0314 | 561 | 1.1237 | 1.1304 | 113 | 1.1377 | 1.0381 | 185 | ||||
SC_gEUD75% | N/A | N/A | N/A | 1.1157 | 1.0660 | 214 | 1.1572 | 0.9809 | 546 | 1.1264 | 1.0994 | 113 | N/A | N/A | N/A | ||||
SC_gEUD70% | 1.1068 | 1.3535 | 427 | N/A | N/A | N/A | 1.1661 | 0.9834 | 538 | 1.1215 | 1.0972 | 120 | 1.1407 | 0.9891 | 183 | ||||
SC_gEUD60% | 1.1327 | 1.4304 | 252 | N/A | N/A | N/A | N/A | N/A | N/A | N/A | N/A | N/A | N/A | N/A | N/A |
DV, dose-volume; SC_gEUD90%, 90% of the gEUD value of the spinal cord calculated from DV plan, and so on; N/A, not available; CI, conformity index; HI, homogeneity index; Dmean, mean dose.
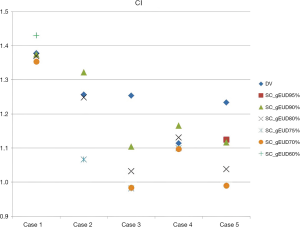
The HI for each individual plan was shown in Table 4 and Figure 4. As to the homogeneity of PTV, DV-gEUD plans performed slightly worse than the DV plans within the same case along with the stepwise stricter Max EUD constraint of SC used. Regarding the HI in all the five DV plans and respective DV-gEUD plans adopting SC_gEUD80% as Max EUD constraint of SC, the mean of HI was higher in the group of DV-gEUD plans with statistical significance (1.1099 vs. 1.1256, P=0.01), indicating poorer homogeneity.
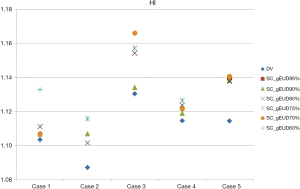
Even though single biologically based gEUD objective function for the SC was added alone on the DV plan for further optimization, improvement of the performance of contralateral parotid gland sparing by reducing the Dmean could be obtained by this optimization approach as an additional benefit. The detailed data regarding Dmean of the contralateral parotid gland for all DV and DV-gEUD plans were shown in Table 4 and Figure 5.
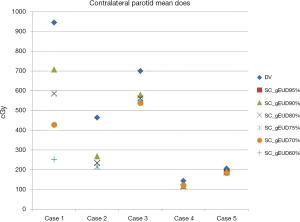
The results of statistical analyses of dosimetric parameters between groups of original DV plans and respective DV-gEUD plans adopting SC_gEUD90% and SC_gEUD80% as SC Max EUD constraint were presented in Table 5.
Table 5
Dosimetric parameter | DV | SC_gEUD90% | SC_gEUD80% | P value | |
---|---|---|---|---|---|
DV |
DV |
||||
SC Dmax (cGy) | 412.2±86.2 | 341.7±106.7 | 328.5±108.8 | 0.039 | 0.018 |
Contralateral parotid Dmean (cGy) | 491.0±337.0 | 371.6±257.5 | 335.4±221.2 | 0.055 | 0.072 |
V100% (%) | 97.72±1.08 | 96.90±1.33 | 95.56±2.20 | 0.136 | 0.053 |
HI | 1.1099±0.0160 | 1.1210±0.0149 | 1.1256±0.0210 | 0.069 | 0.010 |
CI | 1.2472±0.0939 | 1.2163±0.1236 | 1.1635±0.1447 | 0.518 | 0.178 |
DV, dose-volume; gEUD, generalized equivalent uniform dose; SC Dmax, the maximum dose of spinal cord;V100%, the percentage of the target volume receiving the prescription dose; Dmean, mean dose; HI, homogeneity index; CI, conformity index; SC_gEUD90%, 90% of the gEUD value of the spinal cord calculated from DV plan; SC_gEUD80%, 80% of the gEUD value of the spinal cord calculated from DV plan; N/A, not available; SC, spinal cord.
Discussion
Re-irradiation is recognized as a tolerable and effective treatment option for recurrent HNC in the era of sophisticated modern RT technique. The use of re-irradiation is growing and to make every effort to generate an acceptable plan obtaining optimal tumor coverage without jeopardizing the surrounding previously irradiated OARs would be the most challenging and urgent goal. VMAT is considered as a promising and potential ideal technique to apply in such a clinical dilemma. Osborn (7) reviewed the relevant planning and clinical studies regarding the VMAT for HNC concluded that dual arc VMAT could provide the theoretical benefit as similar conformity, better homogeneity and improved sparing of OARs along with shortened treatment time and less MUs compared to IMRT, but also pointed out that more clinical data on the acute and late toxicities is needed to further evaluate the efficacy of VMAT. Dogan (18) conducted a study comparing head and neck VMAT plans using Pinnacle3® SmartArc with either physical DV constraints or gEUD-based approach, and showed that the biological optimization method could further yield 55% reduction in near-to-maximum SC dose, and 35% reduction in mean parotid dose.
To our knowledge, this is the first planning study with primary aim to assess the feasibility and to investigate the potential benefit of incorporating biologically based gEUD objective function in the optimization process of VMAT plans for cases with recurrent HNC treated with re-irradiation. The results of our experience showed that the overall plan quality was further optimized with using the 90% of the SC gEUD calculated from the original DV plan (SC_gEUD90%) to set the added Max EUD constraint by 17.1% reduction of the Dmax of SC in DV-gEUD plan with non-inferior target coverage, similar conformity and slightly poorer homogeneity which was in line with results of other gEUD-based optimization studies (10-13,15,16). It should be noted that the constraint used with respect to SC sparing in particular is quite challenging in our study due to that myelopathy is a devastating complication in the re-irradiation setting, and the method we recommended by additionally setting Max EUD constraint could achieve desirable extremely low dose of it when prevention of myelopathy is of paramount importance. Although DV optimization alone might meet acceptable criteria, further trivial improvement depends on repetitive DV parameters adjustment by trial and error, planner’s experience and time-consuming repeated iterations. Combined with biological optimization offers an efficient and feasible way to facilitate this process for improving overall plan quality in complicated cases. Therefore, our proposed approach is worthy of implementation in the light of these advantages.
As to the result regarding the reduction in the Dmean of contralateral parotid gland by adopting the sole Max EUD constraint of the SC combined with multiple DV objectives in our work, the influence of biological optimization on finely tuning the DVHs resulting in further dose distribution sculpting was demonstrated. Since that the definition of EUD allows for a certain freedom in shaping the dose distribution, EUD constraint is less restrictive than multiple DV constraints and could offer an inherent trade-off between different dose levels, allowing controlled violations for some DV constraints while over-fulfilling other constraints to attain the result of an overall better dose distribution (22).
A phantom study (17) using gEUD objective in the Photon Optimizer of Varian Eclipse TPS to optimize VMAT plans published by Fogliata et al. investigated the interplay of the parameter ɑ selected and OAR sparing. Their work showed that gEUD is a powerful objective tool to improve the OAR sparing without compromising the target coverage and homogeneity when applied with a proper ɑ value selected according to the target/OAR geometry and structure seriality. We adopted single ɑ value of 20 in our study based on two reasons. First, SC is regarded as typical serial organ, and large ɑ value forces the high dose region on DVH. Moreover, the Dmax in the OAR was minimized effectively with ɑ values up to 20, while further increasing ɑ to 40 provided no additional improvement.
The results of case 5 in our study indicated that the distance between OAR and target is a key factor impacting greatly on the extent of benefit provided by this gEUD-assisted optimization process and also confirmed the finding of trade-off between OAR sparing for high doses and target coverage in addition to homogeneity from Fogliata’s phantom study (17). The clinicians need to judge the worthiness of this trade-off with caution in such complicated cases.
Be different from the study design either pure gEUD based used by Dogan, or hybrid method with opposite sequence, namely, initial biological optimization followed by further modification with DV optimization proposed by Guo (10), we simplified the approach proposed by Lee (11) that staring with a DV-generated plan followed by adding gEUD-based optimization with single Max EUD constraint for a chosen OAR instead of multiple EUD objectives applied in their work. Owing to the fact that biological optimization was developed and could be chosen on commercial TPSs for a decade, it is still not popular and unfamiliar to most radiation oncology professionals, a minimal learning curve is expected to incorporate the biological optimization into clinical practice by using this method.
There were several limitations of this study. First, the issue of priority/weight during optimization process related to personal experience and preference might affect the dosimetric outcome. Second, biological evaluation tools such as TCP/NTCP were not used to replace physical indices with the reason as much more challenging task will be necessary to validate such biological models and parameters that attempt to describe treatment outcomes observed clinically in spite of the potential benefits of it. Moreover, we adopted a fixed ɑ parameter of 20 without testing the influence of varied parameter ɑ value. Since that the gEUD (ɑ) value depends on the DVH shape, and there is no published data on gEUD (ɑ) tolerance levels for specific organs. This makes more difficult a correct use of the gEUD objective, once applied the proper organ-specific ɑ value. Our study recommended a useful method for reference in a re-irradiation setting of HNC. Further clinical studies evaluating the patient toxicity related to gEUD and the ɑ parameter for the most important critical structures would be needed for a more biologically conscious use of the gEUD-based optimization. For actual implementation in daily treatment, some factors should also be considered such as patient-specific quality assurance (QA) procedures, MUs and verification of delivery accuracy. Several studies (24,25) reported on the methods for an accurate assessment of the delivered dose on different SC sections and planning techniques to spare doses to the SC, which is considerably important in a re-irradiation setting. We may need further investigations to clarify these issues.
In conclusion, we proposed and proved the feasibility of a simple and efficient way to integrate the biological and physical constraints in the DV-based plan by adopting a sole Max EUD objective for SC with SC_gEUD90% recommended for further biological optimization in order to produce better plan quality in the re-irradiation setting using VMAT for HNC to diminish the risk of myelopathy.
More studies dedicated to a better understanding of the correlation between the ɑ parameter and the OAR radiobiology would be warranted.
Acknowledgments
Funding: None.
Footnote
Conflicts of Interest: All authors have completed the ICMJE uniform disclosure form (available at http://dx.doi.org/10.21037/tro.2018.08.01). The authors have no conflicts of interest to declare.
Ethical Statement: The authors are accountable for all aspects of the work in ensuring that questions related to the accuracy or integrity of any part of the work are appropriately investigated and resolved.
Open Access Statement: This is an Open Access article distributed in accordance with the Creative Commons Attribution-NonCommercial-NoDerivs 4.0 International License (CC BY-NC-ND 4.0), which permits the non-commercial replication and distribution of the article with the strict proviso that no changes or edits are made and the original work is properly cited (including links to both the formal publication through the relevant DOI and the license). See: https://creativecommons.org/licenses/by-nc-nd/4.0/.
References
- Brockstein B, Haraf DJ, Rademaker AW, et al. Patterns of failure, prognostic factors and survival in locoregionally advanced head and neck cancer treated with concomitant chemoradiotherapy: a 9-year, 337-patient, multiinstitutional experience. Ann Oncol 2004;15:1179-86. [Crossref] [PubMed]
- Benson R, Giridhar P, Venkatesulu BP, et al. Re-irradiation for head and neck squamous cell carcinoma. J Egypt Natl Canc Inst 2017;29:1-9. [Crossref] [PubMed]
- Bots WTC, van den Bosch S, Zwijnenburg EM, et al. Reirradiation of head and neck cancer: Long-term disease control and toxicity. Head Neck 2017;39:1122-30. [Crossref] [PubMed]
- Wong SJ, Bourhis J, Langer CJ, et al. Retreatment of recurrent head and neck cancer in a previously irradiated field. Semin Radiat Oncol 2012;22:214-9. [Crossref] [PubMed]
- Lee N, Chan K, Bekelman JE, et al. Salvage re-irradiation for recurrent head and neck cancer. Int J Radiat Oncol Biol Phys 2007;68:731-40. [Crossref] [PubMed]
- Teoh M, Clark C, Wood K, et al. Volumetric modulated arc therapy: a review of current literature and clinical use in practice. Br J Radiol 2011;84:967-96. [Crossref] [PubMed]
- Osborn J. Is VMAT beneficial for patients undergoing radiotherapy to the head and neck? Radiography 2017;23:73-6. [Crossref] [PubMed]
- Niemierko A. Reporting and analyzing dose distributions: a concept of equivalent uniform dose. Med Phys 1997;24:103-10. [Crossref] [PubMed]
- Niemierko A. A generalized concept of equivalent uniform dose (EUD). Med Phys 1999;26:1100.
- Guo C, Zhang P, Gui Z, et al. An Efficient Method for Improving the Dose-Volume-Based Optimization Plan Quality. IEEE Access 2017;5:7520-31.
- Lee TF, Ting H, Chao P, et al. Dosimetric advantages of generalised equivalent uniform dose-based optimisation on dose–volume objectives in intensity-modulated radiotherapy planning for bilateral breast cancer. Br J Radiol 2012;85:1499-506. [Crossref] [PubMed]
- Mihailidis DN, Plants B, Farinash L, et al. Superiority of equivalent uniform dose (EUD)-based optimization for breast and chest wall. Med Dosim 2010;35:67-76. [Crossref] [PubMed]
- Feng Z, Tao C, Zhu J, et al. An integrated strategy of biological and physical constraints in biological optimization for cervical carcinoma. Radiat Oncol 2017;12:64. [Crossref] [PubMed]
- Hartmann M, Bogner L. Investigation of intensity-modulated radiotherapy optimization with gEUD-based objectives by means of simulated annealing. Med Phys 2008;35:2041-9. [Crossref] [PubMed]
- Semenenko VA, Reitz B, Day E, et al. Evaluation of a commercial biologically based IMRT treatment planning system. Med Phys 2008;35:5851-60. [Crossref] [PubMed]
- Wu Q, Djajaputra D, Liu HH, et al. Dose sculpting with generalized equivalent uniform dose. Med Phys 2005;32:1387-96. [Crossref] [PubMed]
- Fogliata A, Thompson S, Stravato A, et al. On the gEUD biological optimization objective for organs at risk in Photon Optimizer of Eclipse treatment planning system. J Appl Clin Med Phys 2018;19:106-14. [Crossref] [PubMed]
- Dogan N, Wu Y. SU-E-T-582: Assessment of Improved Critical Structure Sparing Using Biologically-Based Optimization for Volumetric Modulated Arc Therapy of Head and Neck Cancer. Med Phys 2012;39:3839-40. [Crossref] [PubMed]
- Kirkpatrick JP, van der Kogel AJ, Schultheiss TE. Radiation dose–volume effects in the spinal cord. Int J Radiat Oncol Biol Phys 2010;76:S42-9. [Crossref] [PubMed]
- Sminia P, Oldenburger F, Slotman BJ, et al. Re-irradiation of the human spinal cord. Strahlenther Onkol 2002;178:453-6. [Crossref] [PubMed]
- Strojan P, Corry J, Eisbruch A, et al. Recurrent and second primary squamous cell carcinoma of the head and neck: when and how to reirradiate. Head Neck 2015;37:134-50. [Crossref] [PubMed]
- Allen Li X, Alber M, Deasy JO, et al. The use and QA of biologically related models for treatment planning: Short report of the TG-166 of the therapy physics committee of the AAPM. Med Phys 2012;39:1386-409. [Crossref] [PubMed]
- Feuvret L, Noël G, Mazeron JJ, et al. Conformity index: a review. Int J Radiat Oncol Biol Phys 2006;64:333-42. [Crossref] [PubMed]
- Chen CC, Lee CC, Mah D, et al. Dose sparing of brainstem and spinal cord for re-irradiating recurrent head and neck cancer with intensity-modulated radiotherapy. Med Dosim 2011;36:21-7. [Crossref] [PubMed]
- Stoiber EM, Schwarz M, Debus J, et al. Regional cumulative maximum dose to the spinal cord in head-and-neck cancer: Considerations for re-irradiation. Radiother Oncol 2013;106:96-100. [Crossref] [PubMed]
Cite this article as: Lee CH, Jhang ZR, Yeh SA, Chen YL. A planning study for evaluation of feasibility and benefit of generalized equivalent uniform dose-assisted optimization in volumetric-modulated arc therapy for recurrent head and neck cancer. Ther Radiol Oncol 2018;2:36.